8 Perfusion and Diffusion Limitations in Gas Exchange
Learning objectives
- Differentiate between diffusion and perfusion limitations to gas exchange and describe the effects of exercise and lung disease on these limiting factors.
- Describe how reaction rates with hemoglobin influence the transfer of oxygen.
From the description of Fick’s law of diffusion, gas exchange between the alveoli and blood depends on a maintained diffusion gradient and favorable membrane properties. Gas exchange can become limited if:
- There is an impediment to diffusion across the membrane (e.g., an increase in membrane thickness T or a reduction in surface area A),
OR
- The diffusion gradient across the membrane is reduced (i.e., reduced P1−P2). This can occur if perfusion is too low.
Identifying whether deranged blood gases are due to diffusion or perfusion limitations can help in the diagnosis of an underlying pathological issue, so we will look at them here.
Diffusion Versus Perfusion Limitations
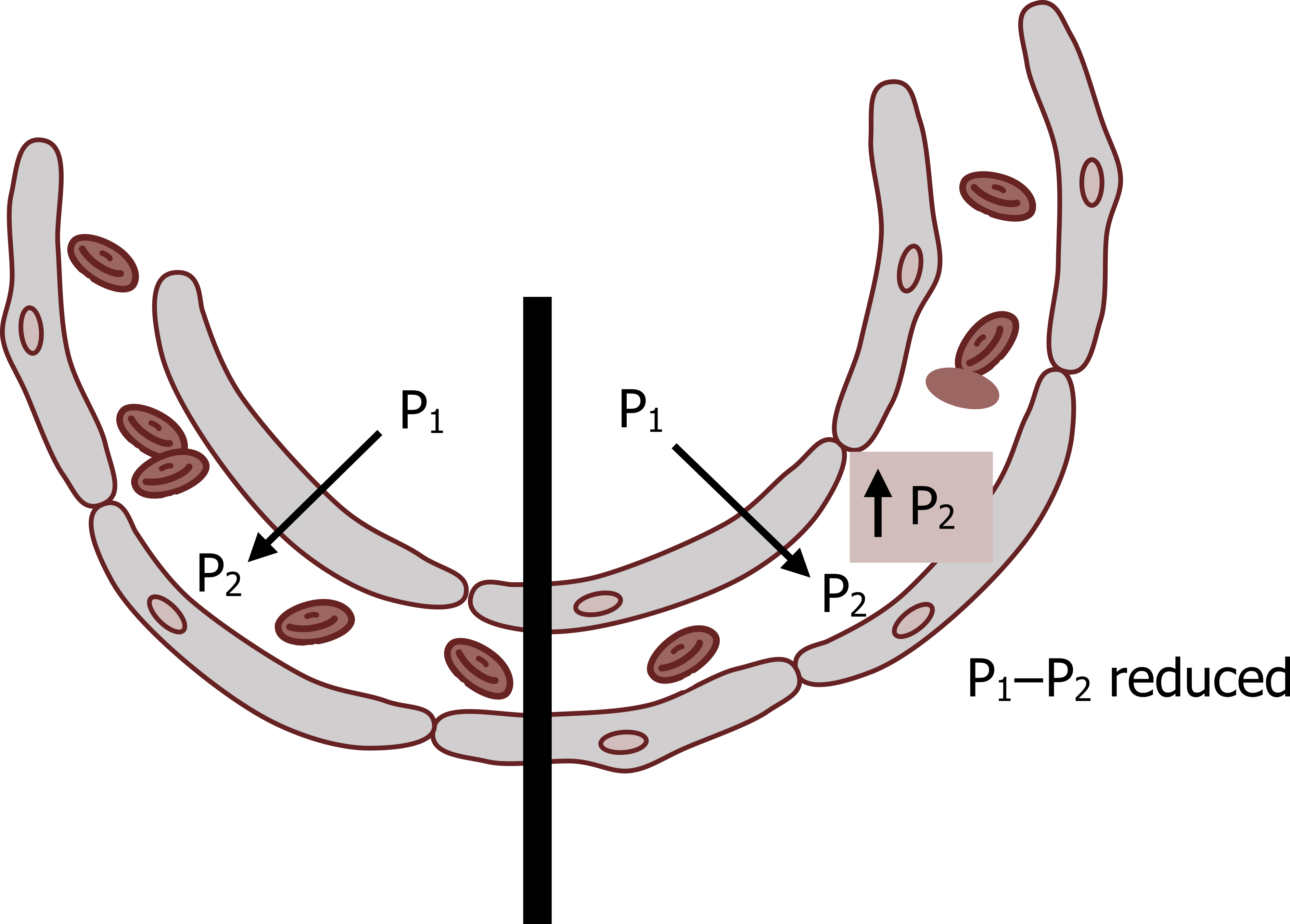
If a sufficient diffusion gradient exists across a membrane, then the rate of transfer of gas is primarily dependent on the properties of the membrane (see the left side of the figure 8.1). The transfer of gas in this case is referred to as a diffusion limitation.
If gas starts to accumulate on the other side of the membrane, however, the pressure gradient will dissipate and transfer rates become limited (right side of figure 8.1). This is referred to as a perfusion limitation, as it is indicative of low blood flow that is insufficient to “wash away” transferred gas, keep blood gas partial pressure low, and maintain the diffusion gradient.
We can illustrate these diffusion and perfusion limitations with the behavior of two nonphysiological gases transferring from the alveolus to the bloodstream.
Carbon monoxide is well known for its affinity for hemoglobin. When CO passes across the membrane it rapidly binds to hemoglobin and is thus removed from solution. This removal from solution maintains the pressure gradient across the membrane. So with a maintained pressure gradient the major impediment to CO transfer is diffusion across the membrane. Therefore CO transfer is referred to as diffusion limited and dependent on the properties of the membrane. (Consequently CO is used in diffusion limitation testing (DLCO) in pulmonary function labs.)
Nitrous oxide, alternatively, does not bind with hemoglobin at all, so its arterial partial pressure rises rapidly as it stays in solution. So maintaining the pressure gradient is dependent on how quickly the transferred nitrous oxide is washed away by blood flow. Because of this, nitrous oxide is referred to as perfusion limited.
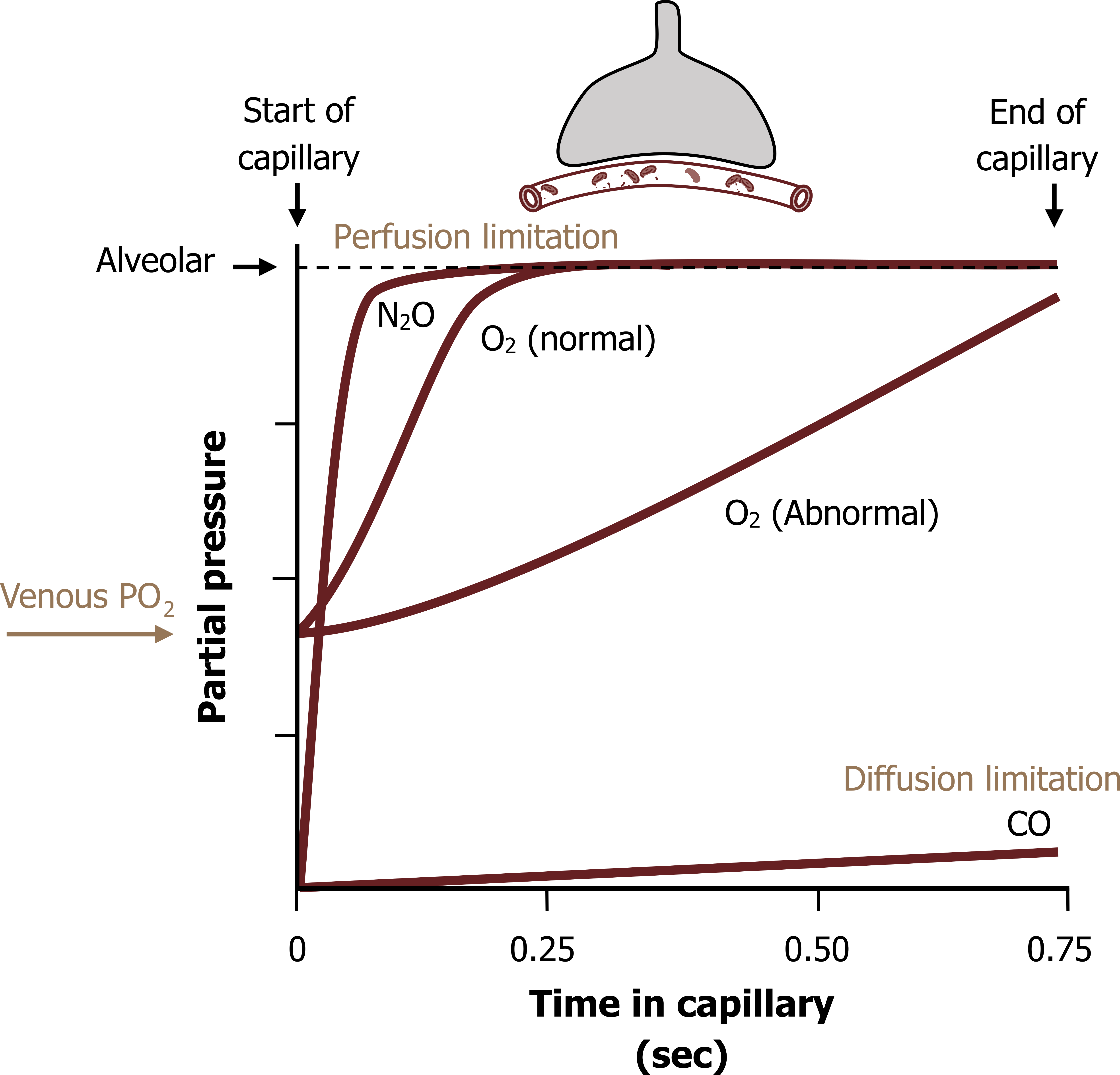
So while our two nonphysiological gases provide good examples of diffusion and perfusion limitations, let us see how oxygen behaves.
Figure 8.2 shows the arterial partial pressures of gases against time in the capillary—think of it as transit time; it takes 0.75 seconds for blood to pass the alveolar surface.
The blood partial pressure of CO rises very little along the capillary as it is rapidly binding to hemoglobin (Hb) (figure 8.2), the pressure gradient is maintained, and the CO is only limited by the membrane; it exhibits diffusion limitation.
The blood partial pressure of nitrous oxide, alternatively, rapidly rises (figure 8.2), and the transfer of NO becomes reliant on the rate of perfusion to maintain the gradient and gas transfer (i.e., it is perfusion limited).
The results for O2 fall much closer to the perfusion limitation (NO) line than the diffusion limitation line (figure 8.2, O2 normal). Oxygen binds to hemoglobin so the arterial PO2 does not rise as quickly as nitrous oxide, but the binding of O2 is so much less than carbon monoxide it actually demonstrates more perfusion, rather than diffusion limitation.
The transfer of O2 is also hampered by having to start off at venous partial pressures (40 mmHg), compared to our test gases that start off at zero. Consequently the initial pressure gradient is less. Despite this, the arterial partial pressure of oxygen equilibrates with alveolar pressures within 0.25 seconds (i.e., a third of the distance around the capillary). With the blood still having another 0.5 seconds in the capillary, this provides a large reserve time.
This reserve time is often eaten into in some disease states (figure 8.2, abnormal); if for example a diffusion problem arises, such as thickening of the membrane, then that extra 0.5 seconds, or last 2/3 seconds of the transit time around the alveolus, can still allow alveolar and arterial PO2s to equilibrate. The patient may still show normal oxygen pressures until they exercise, during which the velocity of pulmonary blood flow increases and transit time is reduced; you can see from figure 8.2 if transit time is reduced to 0.5 seconds then arterial PO2 will not equilibrate with alveolar values in the abnormal lung.
While in the normal state the transfer of oxygen is perfusion limited, in lung diseases that affect the surface area or membrane thickness of the gas exchange surface, the transfer of oxygen may become diffusion limited. Being able to measure the transfer of gas into the blood provides a valuable diagnostic tool. This is what we will look at here, not only because of its clinical pertinence, but also because it summarizes some physiological principles.
Testing the Diffusion Capacity of the Lung
The gas used to test the diffusion capacity of the lung is carbon monoxide. Why? Because as you have seen, carbon monoxide is diffusion limited, so any change in membrane characteristics will affect its movement into the bloodstream. So fundamentally, we will have the patient inhale a little carbon monoxide and hold their breath for a few seconds. During the breath-hold some CO will move into the bloodstream—the greater the disease (diffusion limitation), the more will stay in the lung. As the patient breathes out, the exhaled carbon monoxide (i.e., that not transferred) is measured. The difference between the amount inhaled and the amount returned in the exhalation is the amount that crossed into the blood. The more CO that comes back in the exhalation, the less that crossed into the blood and the worse diffusion limitation is.
Let us look at this more formally.
The transfer factor is primarily dictated by the factors within Fick’s law of diffusion. Because we cannot independently measure membrane area and thickness, we lump these terms and the diffusion coefficient of the gas we are interested in into one term, the diffusing capacity of the lung, or DL (equations below).
Equation 8.1
[latex]\dot{V}_{gas} = \frac{A}{T} \times D \times (P_1 - P_2)[/latex]
↓
Equation 8.2
[latex]\dot{V}_{gas} = D_L \times (P_1 - P_2)[/latex]
↓
Equation 8.3
[latex]D_L = \displaystyle\frac{\dot{V}_{CO}}{P_1 - P_2}[/latex]
↓
Equation 8.4
[latex]D_L = \displaystyle\frac{\dot{V}_{CO}}{P_{A_{CO}}}[/latex]
In the lab test, we are specifically looking at the transfer, or flow, of CO across the membrane, and if we rearrange this equation for transfer factor we see the transfer factor is the flow of CO divided by the pressure gradient of CO (equation 8.4). We can assume that the arterial partial pressure of CO is zero, so our equation for transfer factor ends up as the flow, or transfer, of CO across the membrane, divided by the alveolar partial pressure of CO.
But the movement of gases such as CO, and more physiologically important, oxygen, is also determined by the rate of binding with hemoglobin when it gets into the bloodstream. So our transfer factor has to contain an additional term to account for this. The rate of binding to hemoglobin is determined by two factors; first, the affinity of the gas for hemoglobin, denoted here by theta, and second, by the amount of hemoglobin present in the capillary, denoted as Vc, or capillary volume.
Equation 8.5
[latex]\displaystyle\frac{1}{D_L} = \displaystyle\frac{1}{D_M} + \displaystyle\frac{1}{\theta \times V_c}[/latex]
So now if we rename our initial value of DL that described the factors associated with the membrane as DM and add our term to account for binding with hemoglobin (equation 8.5), the sum of these two gives a more complete description of transfer, or DL. This more complete term now reflects that the transfer of gas is not solely dependent on membrane properties.
This provides a relatively simple and powerful diagnostic technique to assess disease stage and reduced function of the lung as a gas exchange organ.
Summary
So we have seen the two major factors that affect the rate of gas transfer across the lung: the rate of diffusion that is determined by the characteristics of the membrane (as described in Fick’s law of diffusion), and also the rate of perfusion, which involves the rate of blood flow, volume, and binding affinity with hemoglobin. Diffusion limitation is really a description of the impediment caused by the membrane with a constant partial pressure gradient; and perfusion limitation describes whether the partial pressure gradient is being maintained.
References, Resources, and Further Reading
Text
Levitsky, Michael G. “Chapter 6: Diffusion of Gases and Interpretation of Pulmonary Function Tests.” In Pulmonary Physiology, 9th ed. New York: McGraw Hill Education, 2018.
West, John B. “Chapter 3: Diffusion—How Gas Gets Across the Blood–Gas Barrier.” In Respiratory Physiology: The Essentials, 9th ed. Philadelphia: Wolters Kluwer Health/Lippincott Williams and Wilkins, 2012.
Widdicombe, John G., and Andrew S. Davis. “Chapter 4.” In Respiratory Physiology. Baltimore: University Park Press, 1983.
Figures
Figure 8.1: Diffusion and perfusion limitations. Grey, Kindred. 2022. CC BY 4.0. Added red blood cells by Lucas Helle from Noun Project. CC BY 3.0. https://archive.org/details/8.1_20220125
Figure 8.2: Transfer of gases from alveolus to capillary. Grey, Kindred. 2022. CC BY 4.0. Added red blood cells by Lucas Helle from Noun Project. CC BY 3.0. https://archive.org/details/8.2_20220125