2 Mechanics of the Lungs
Learning objectives
- Describe the changes in pleural and alveolar pressures and lung volume caused by contraction of respiratory muscles over the respiratory cycle.
Fundamentals of Gas Movement
This chapter describes the mechanisms by which air is moved into and out of the lung, or pulmonary mechanics.
Before we get into the details of how we breathe in, let me make sure we are all on the same page by going right back to basics, so bare with me or skip ahead if you are happy with pressure, volume, and flow.
To get air to move into the lungs we need to generate a pressure differential; that is the pressure inside the lungs must be lower than the pressure outside (i.e., atmospheric pressure), so that air moves down the pressure gradient into the lungs.
The low pressure inside the lungs is generated by increasing lung volume; bigger volume means fewer molecules in the same space, and therefore lower pressure (go back and revisit Boyle’s law if needed).
The basis of inspiration is lowering lung pressure below atmospheric pressure, so that atmospheric pressure pushes air down the airways until pressure equilibrates. So the fundamental first step is, how do we increase lung volume?
To understand the mechanics of breathing we have to deal with two concepts: first how the action of the respiratory muscles increases thoracic volume, and second (and more complex) we need to understand the interaction of the lungs and the thoracic wall.
Let us deal with the respiratory muscles and expansion of the thorax first.
Changing Thoracic Volume
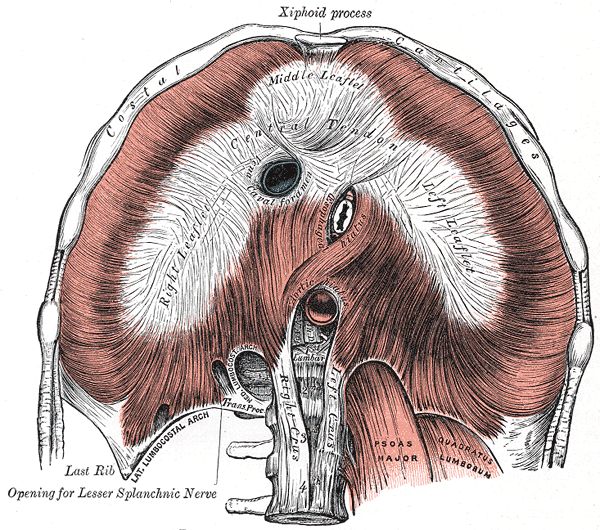
The muscle that generates the greatest change in thoracic volume (and thereby the greatest contribution to breathing) is the diaphragm (figure 2.1). Separating the thoracic and abdominal cavities, this sheetlike muscle forms a dome shape in the relaxed state that encroaches into the thorax. This sheet is formed of three sections, the anterior portion originating at the ribs and sternum, and the posterior portion originating on the vertebrae. These are connected by the central portion that is comprised of a tendon sheet.
It is worth a quick reminder that while controlling a visceral organ and performing a homeostatic function, the diaphragm and the other respiratory muscles are skeletal muscle and have the force-generation characteristics of such. As well as being under reflex control, it can also be controlled voluntarily (such as during speech).
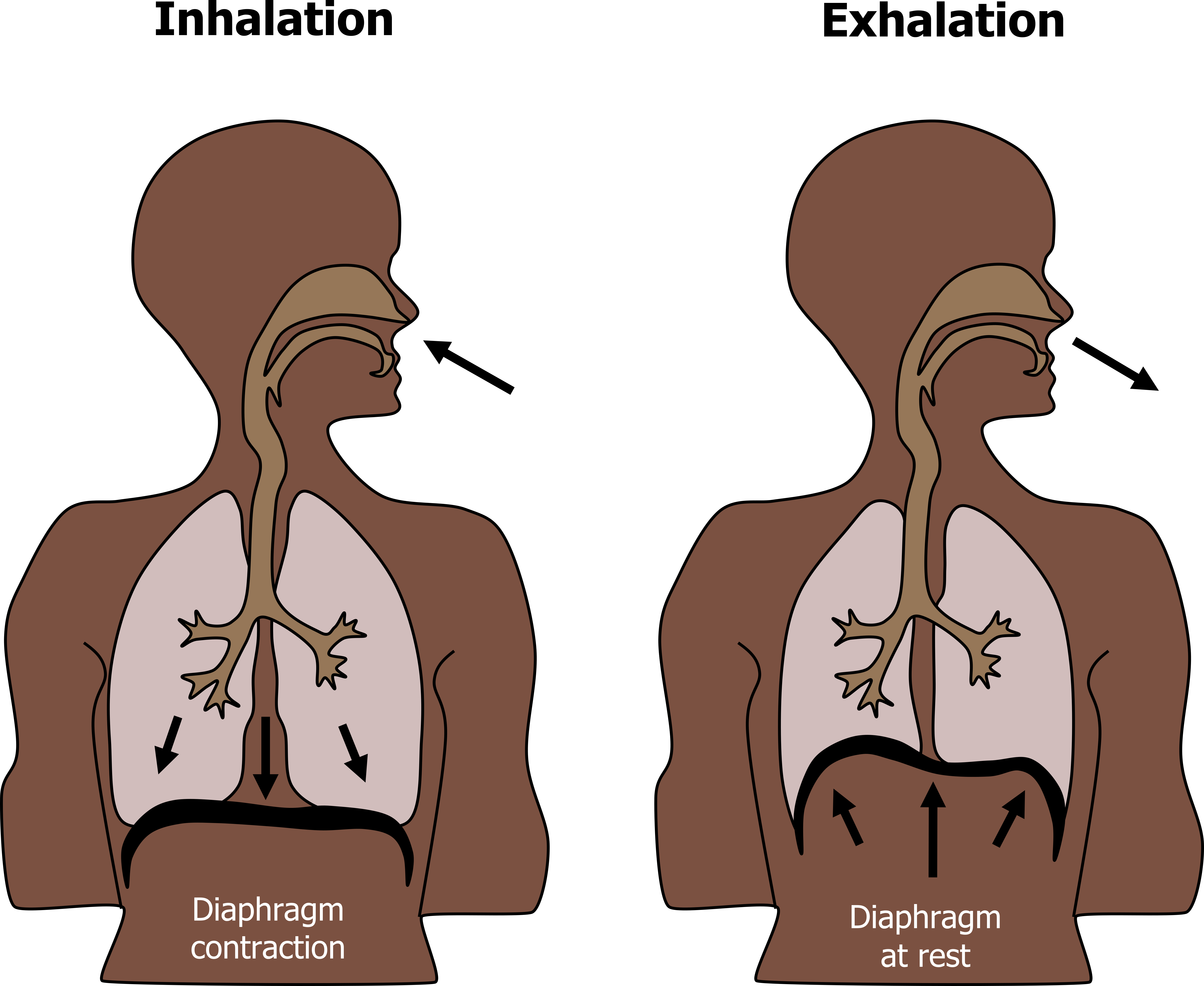
Activation of the phrenic nerve stimulates the diaphragm and generates inspiration. Upon stimulation the contracting diaphragm flattens out, descending toward the abdomen. As it does so the thoracic volume increases, and consequently thoracic pressure falls. When thoracic pressure falls below atmospheric pressure, air moves down the generated pressure gradient and enters the lung. Note that this increase in thoracic volume comes at the expense of the abdominal volume, and abdominal contents can be compressed during inspiration. The diaphragm may descend as much as 10 cm, but a descent of 1 cm is sufficient to provide tidal breathing (figure 2.2).
When phrenic nerve activity stops, the diaphragm relaxes and returns to its resting dome-like position; this is aided by the recoil of the expanded lung and the decompression of the abdominal contents. The return to the resting position reduces thoracic volume and increases thoracic pressure above atmospheric pressure and air exits the lung down the reversed pressure gradient.
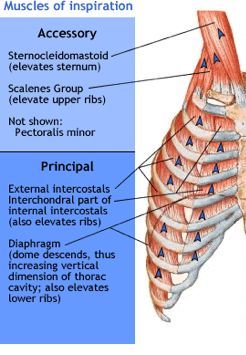
During inspiration the thoracic volume is also increased by the action of the external intercostal muscles. Controlled by the intercostal nerve, contraction of the external intercostals causes the rib cage to rise upward and outward, resulting in an expansion of the thoracic volume in addition to the action of the diaphragm. This action is generated by the oblique positioning of the external intercostals between the ribs, and the sternum and upper ribs are stabilized by simultaneous activation of the scalenus muscles.
During periods of high ventilatory need (or drive) other muscles can contribute to expansion of the rib cage (figure 2.3). These “accessory” muscles assist the external intercostals and include the sternocleidomastoids, the scalenes, and the pectoralis minor. All of these groups allow for a greater thoracic expansion and thus a greater lung volume. Recognizing that a patient is using these muscles to breath is a useful clinical sign; use of these muscles during rest is highly indicative of a raised respiratory effort to cope with an underlying and probably significant problem.
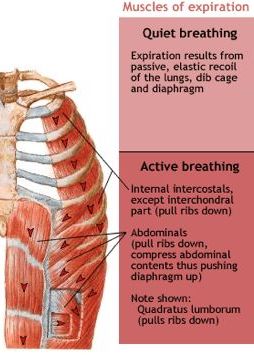
Expiration is generally simpler. The elastic tissue of the lung has been expanded during inspiration, and a little like letting go of a stretch elastic band, the lungs recoil when the inspiratory muscles relax. This recoil reduces lung volume and increases lung pressure above atmospheric pressure and air exits the lung. Depending on the final lung volume achieved during inspiration, recoil of the chest wall may also contribute to expiration.
So during quiet resting breathing, expiration is passive, relying on the expenditure of the stored, potential energy in the elastic lung tissue. However, when ventilation needs to be increased, such as during exercise, this process is too slow, and this passive process needs some active help in order to increase the rate of breathing. Activation of the internal intercostal muscles draws the rib cage downward to reduce thoracic volume. Thoracic volume is further decreased by contraction of muscles surrounding the abdomen; these increase abdominal pressure and help push the diaphragm upward (figure 2.4).
How the Lungs Move with the Chest Wall
Now having dealt with the expansion of the thoracic cage, we should look at the relationship between the thoracic wall and the lungs and how the lungs and the inside of the thorax are adhered to each other so when the thoracic wall moves the lungs follow.
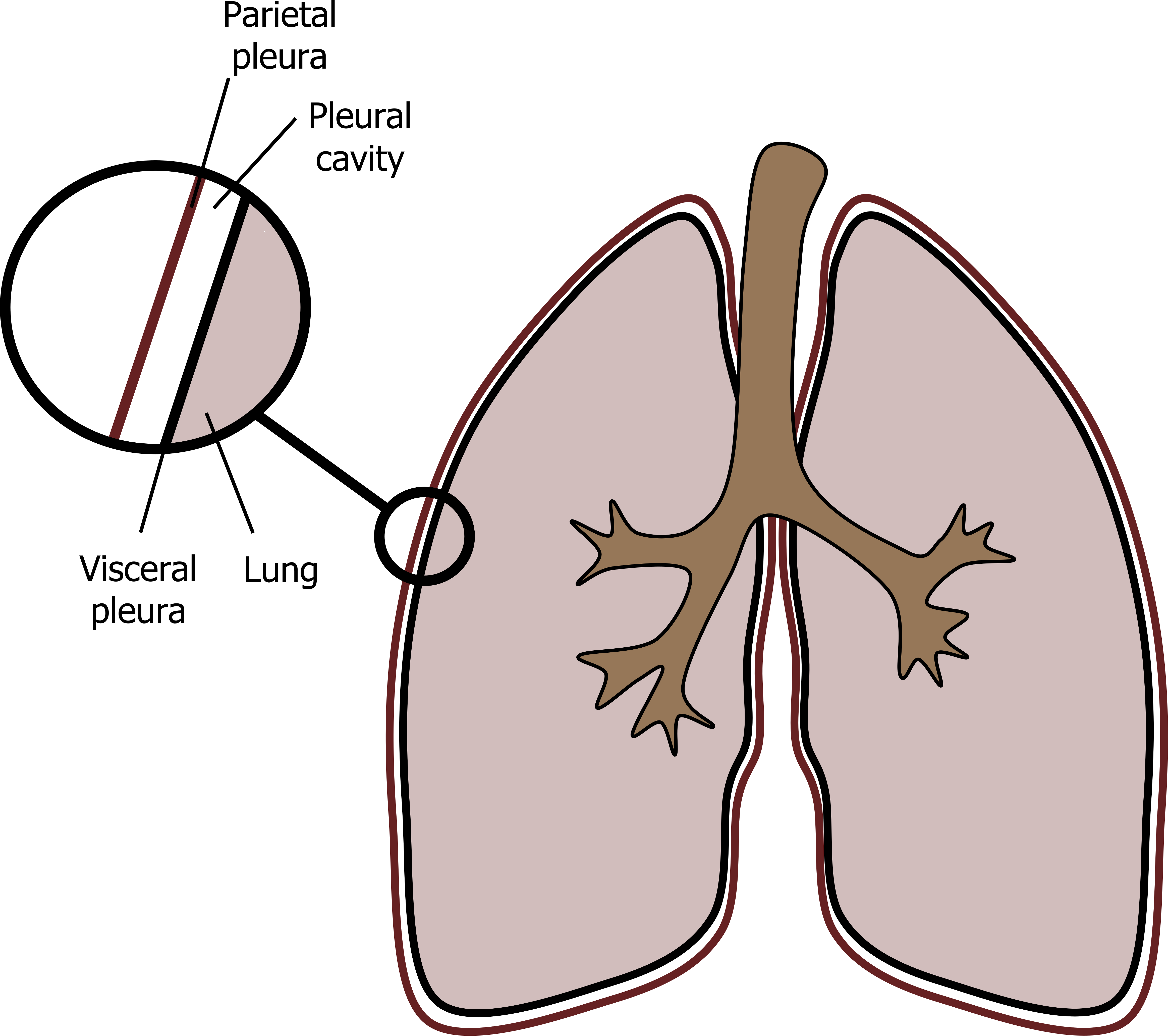
The inside of the thoracic cavity is lined with a membrane, the parietal pleura. The outside of the lungs are lined with a membrane called the visceral pleura. The space between these membranes, the pleural cavity or pleural space, is filled with pleural fluid (figure 2.5). Normally there is only 5–10 mL of pleural fluid to cover all the lung’s external surface. So the fluid layer and the intra-pleural space is extremely thin. When a thin layer of fluid is trapped between two surfaces it exerts surface tension and holds the two surfaces together; if you’ve ever been doing the washing up and trapped a layer of water between too dinner plates you’ll have noticed its difficult to pry the plates apart. It is similar for the pleural membranes, and it is this surface tension that holds the outside of the lungs to the inside of the thorax. We will deal with surface tension in more detail in a later chapter.
We have mentioned the pressure inside the lungs, but now we have to think about the pressure inside the pleural space—called intra-pleural pressure. Even at normal, resting lung volumes the elastic tissue in the lungs is already somewhat stretched, so the lungs have a tendency to recoil, pulling inward. The chest wall, alternatively, has a tendency to spring outward. These opposing movements are prevented by the surface tension in the pleural space and cause a negative intrapleural pressure, that is below atmospheric pressure.
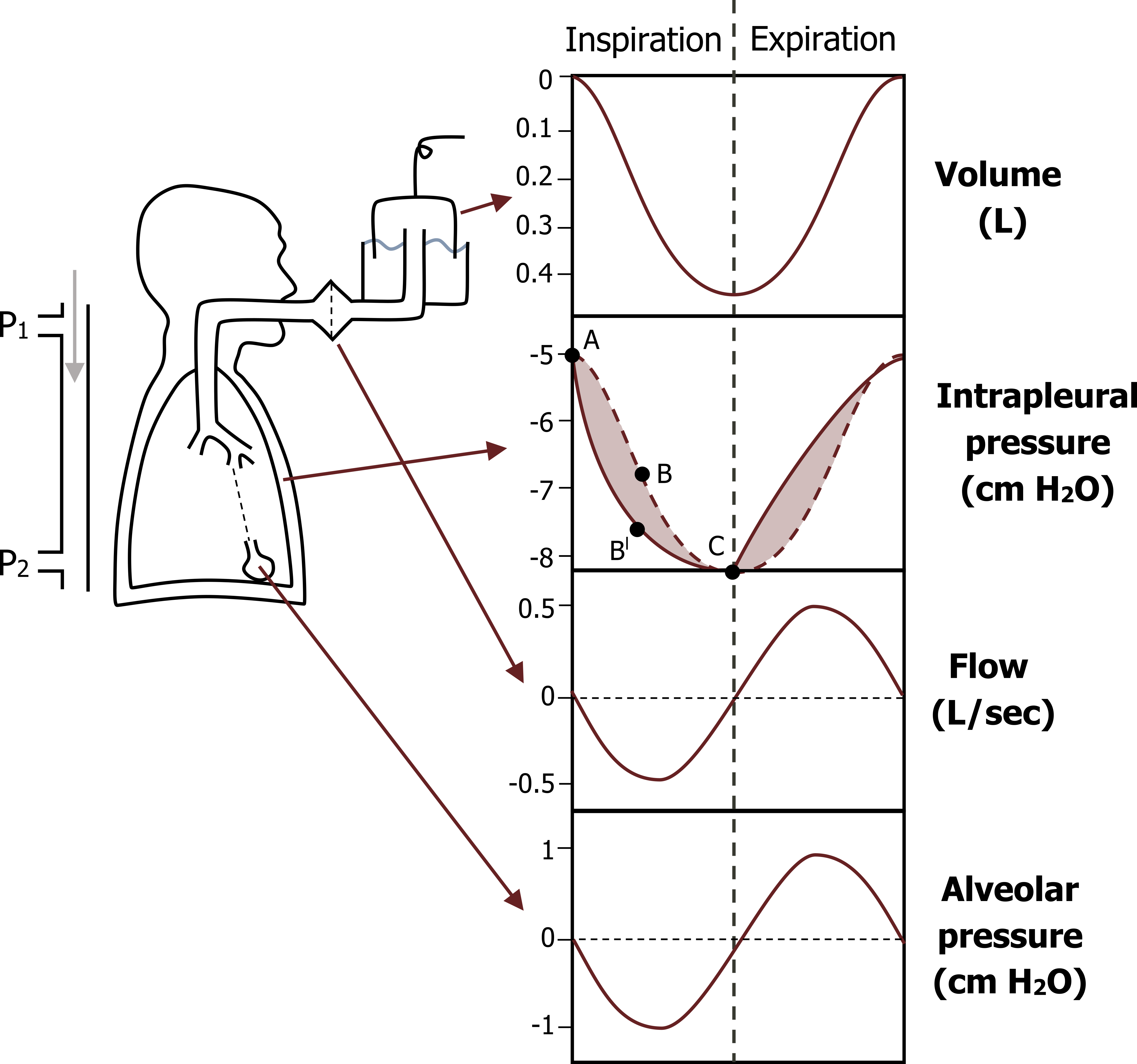
We should now consider what happens to intrapleural and airway pressures during the breathing cycle. First, let us look at the pressures and volumes before inspiration begins (figure 2.6). The intrapleural pressure is slightly negative (−5 cm H2O) due to the recoil of the lung and outward spring of the chest wall. Before the breath starts lung volume is considered zero and flow is also zero (i.e., volume has not changed and there is no movement of air in the airways). Alveolar pressure, the pressure inside the lungs, is also zero, really meaning it is equal to atmospheric pressure.
Look at what happens (figure 2.6) when the respiratory muscles are activated to increase thoracic volume and achieve a breath in. As the thoracic wall moves outward and the diaphragm descends, thoracic volume and therefore lung volume increases. More tension is generated in the stretching elastic tissue of the lungs as the lung expands—and just like stretching an elastic band, the recoil force increases, and the stretching lung now pulls back harder on the pleural space. This causes the intrapleural pressure to become even more negative (−8 cm H2O).
This increase in lung volume (and referring back to Boyle’s law, the pressure of a gas tends to decrease as the volume of the container increases) causes a decrease in pressure in the lung. This is reflected in a decrease in alveolar pressure.
This drop in alveolar pressure generates a pressure differential between the airways and the atmosphere outside—the atmospheric pressure now being greater than the reduced airway pressure causes the flow of air into the airways and toward the alveoli.
Now let us look at these pressures during expiration. At the end of inspiration the lungs are stretched and the recoil force is high. When the activity of the inspiratory muscles stops, the recoil of the lung is unopposed and the lung recoils (a little like letting go of that stretched elastic band). Therefore, in quiet breathing, the process of breathing out is normally passive and relies on the potential energy stored in the lungs’ elastic tissue.
As the lung recoils and returns toward its resting position, the intrapleural pressure becomes less negative and the volume decreases, resulting in a rise in alveolar pressure, as described by Boyle’s law. This rise in alveolar pressure means the pressure gradient is reversed, with pressure inside the lung becoming greater than atmospheric pressure. This reversed pressure differential causes the flow of air from the airways toward the outside—and expiration is achieved.
As already mentioned, this is a passive process that relies on lung recoil, and the expiratory muscles remain inactive during quiet breathing. However, when there is a greater ventilatory demand, such as during exercise or lung disease, the respiratory system cannot wait for this passive and relatively slow process to occur, so the expiratory muscles are activated and thoracic volume (and therefore lung volume) is reduced actively much more quickly; this may cause intrapleural pressure to go positive as the thoracic wall actively pushes on the intrapleural space (and the lungs). This positive pleural pressure during active expiration can have significant ramifications in diseased lungs that we will see later on.
References, Resources, and Further Reading
Text
Levitsky, Michael G. “Chapter 2: Mechanics of Breathing.” In Pulmonary Physiology, 9th ed. New York: McGraw Hill Education, 2018.
West, John B. “Chapter 2: Ventilation—How Gas Gets to the Alveoli.” In Respiratory Physiology: The Essentials, 9th ed. Philadelphia: Wolters Kluwer Health/Lippincott Williams and Wilkins, 2012.
West, John B. “Chapter 7: Mechanics of Breathing—How the Lung Is Supported and Moved.” In Respiratory Physiology: The Essentials, 9th ed. Philadelphia: Wolters Kluwer Health/Lippincott Williams and Wilkins, 2012.
Widdicombe, John G., and Andrew S. Davis. “Chapter 1.” In Respiratory Physiology. Baltimore: University Park Press, 1983.
Figures
Figure 2.1: The diaphragm. Gray, Henry. 1918. Public domain. https://commons.wikimedia.org/wiki/File:Diaphragm_-_2.png
Figure 2.2: Diaphragm positional change. Grey, Kindred. 2022. CC BY 4.0. https://archive.org/details/2.2_20220125
Figure 2.3: Inspiratory muscles of the rib cage. Hafeez, Andeela. 2014. Public domain. https://www.physio-pedia.com/File:949_937_muscles-of-respiration.jpg
Figure 2.4: Expiratory muscles. Hafeez, Andeela. 2014. Public domain. https://www.physio-pedia.com/File:949_937_muscles-of-respiration.jpg
Figure 2.5: The pleural membranes and space. Grey, Kindred. 2022. CC BY 4.0. https://archive.org/details/2.5_20220125
Figure 2.6: The breathing cycle. Grey, Kindred. 2022. CC BY 4.0. https://archive.org/details/2.6_20220125