18 Dyspnea
Learning objectives
- Describe the neural origins of the sensations of air hunger, effort to breathe, and chest tightness, and their prevalence in different disease states.
Occurrence and Forms of Dyspnea
Introduction
Dyspnea is the clinical term for “shortness of breath.” However, the term “dyspnea” no longer describes one sensation but likely includes at least three distinctive sensations associated with breathing that have separate underlying neural mechanisms. This chapter will give you an overview of these sensations and the potential clinical importance of being able to distinguish them.
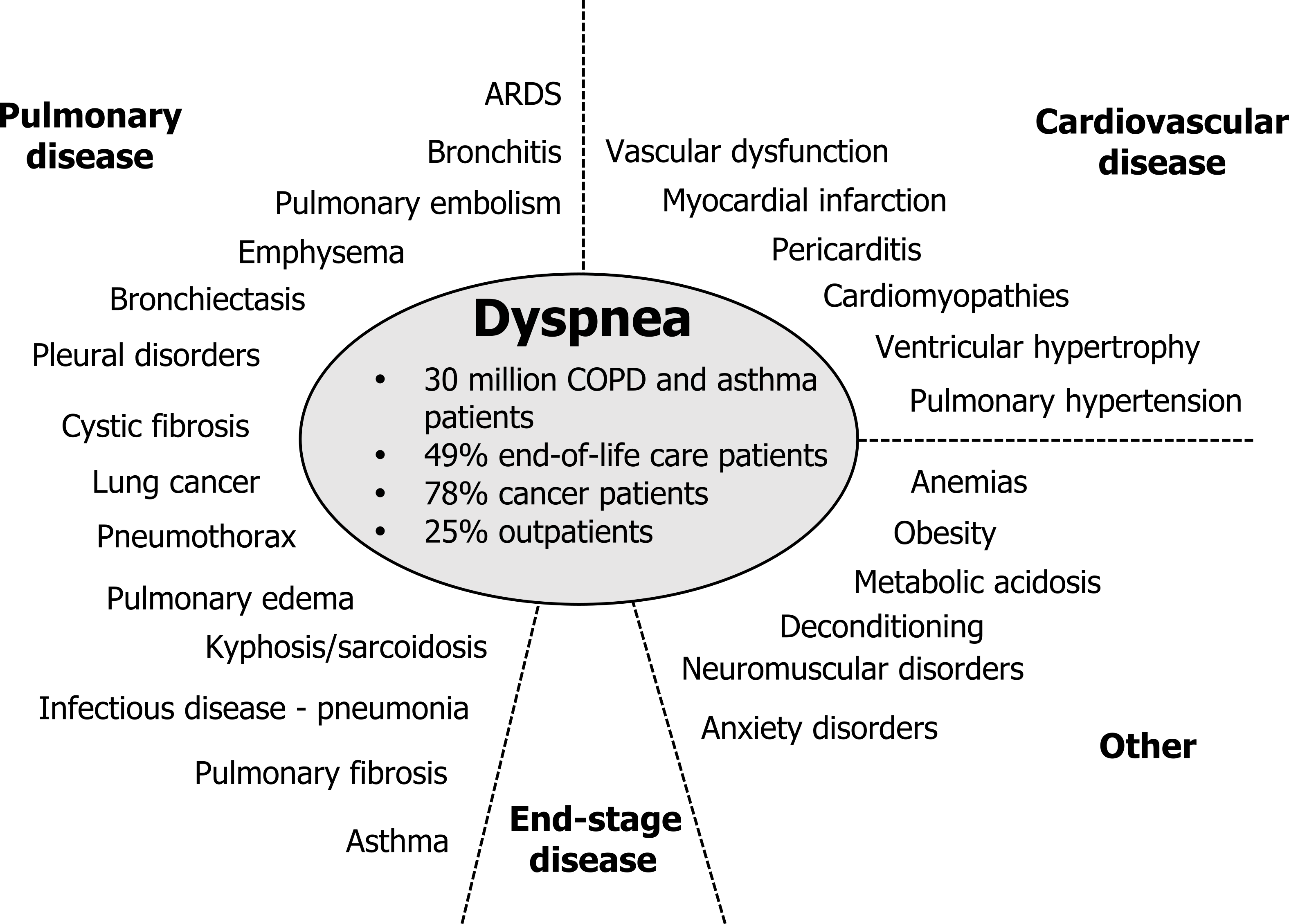
It might be worth putting dyspnea in a clinical context. Like pain, dyspnea can occur across a number of pathological conditions. It is the cardinal symptom of lung disease, but it is highly prevalent in heart diseases as well—in fact it is a more common sign of myocardial infarction in women than the classical symptom of chest pain that is more prevalent in men.
Dyspnea is also a strong predictor of mortality in most heart and lung diseases. As well as cardiopulmonary conditions, dyspnea is also prevalent in other conditions that affect breathing or metabolism, and (see figure 18.1) it is prevalent during end-stage disease where it is as common as pain and forms a significant problem for end-of-life care. Despite its prevalence there are few options for treating this symptom. Unlike pain, there are no specific drugs to reduce this sensation.
Forms of Dyspnea
So now let us look at the distinguishable sensations that the term dyspnea encompasses and begin to understand how they differ neurologically (see figure 18.2).
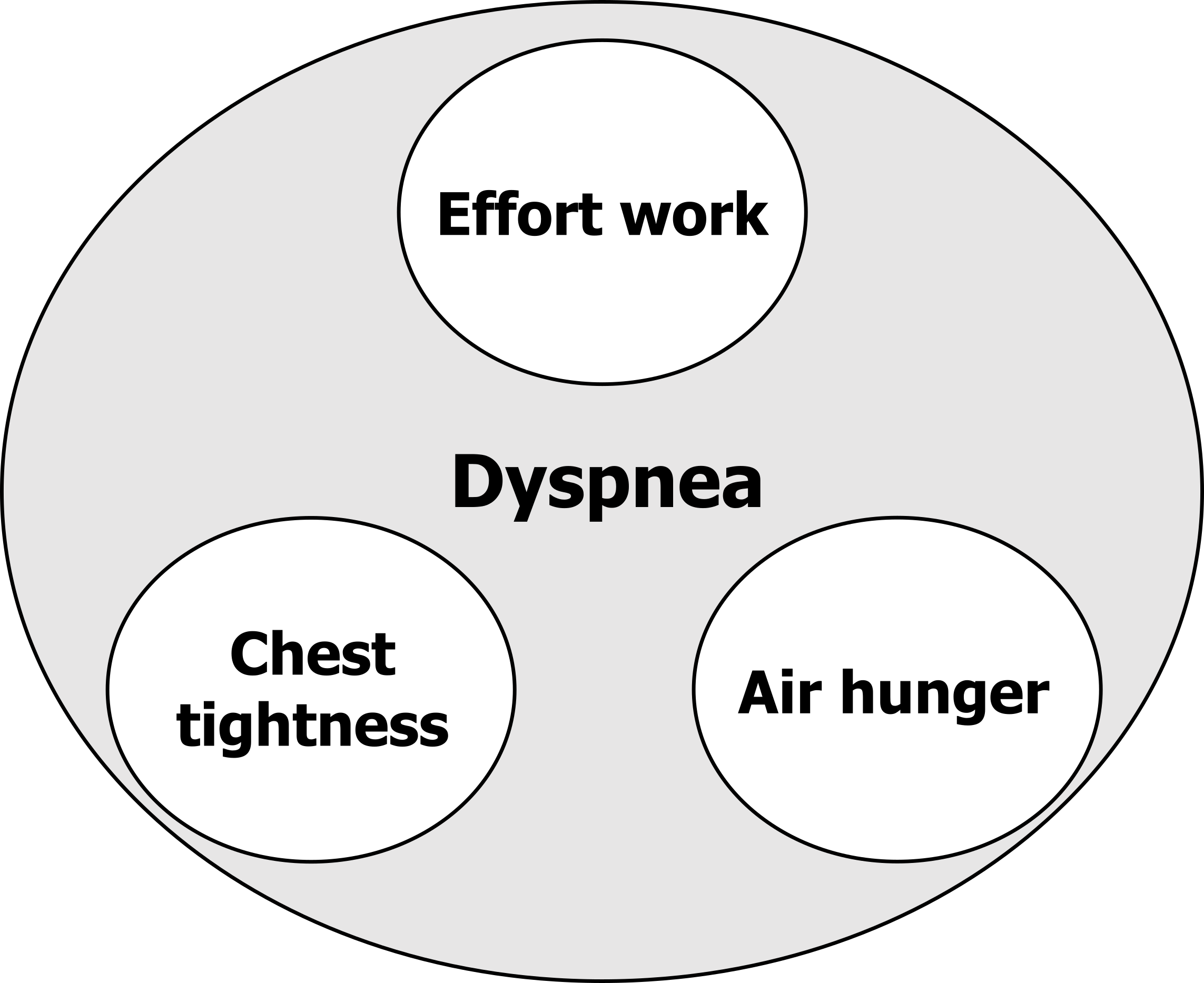
Effort to breathe: The first form of dyspnea is the sensation of work or effort to breathe. The healthy individual is usually unaware of the effort they are putting into breathing until breathing is significantly increased, such as during exercise when ventilation and work of breathing rises. The sensation of the work or effort to breathe is not particularly uncomfortable. If you jogged down the street now you might become more aware of the effort to breathe, but are not disturbed by it.
So where does this sensation come from?
An increase in motor drive is required to activate more tension or movement in any skeletal muscle, including the respiratory muscles. And like other skeletal muscles, such as limb muscles, we believe that the sensation of effort comes from a perception of that increased motor drive. Sensory information from the activated muscles, in our case the respiratory muscles, is thought to generate the sensation of work.
Getting laboratory subjects to report work and effort separately is very difficult, so for our purposes right now, we are grouping what might be two sensations together as one.
Chest tightness: The next form of dyspnea is primarily reported by asthmatic patients during bronchoconstriction. Similar to the sensation of work and effort, tightness was originally thought to arise from the increase in respiratory muscle activity associated with a rise in resistive work of breathing. But in 2002 we showed that “tightness” was unrelated to respiratory effort by removing respiratory muscle activity of bronchoconstricted asthmatics with mechanical ventilation. When we did this, “tightness” persisted, despite the respiratory muscles being inactive. So what does cause tightness? The next best, but so far unproven, alternative is that inflammation of the airways associated with an asthma attack leads to activation of airway irritant (or rapidly adapting) receptors, the afferent activity from which is perceived centrally as tightness.
Air hunger: Air hunger is arguably the most complex and clinically important form. “Air hunger” is the sensation of suffocation and can be described as a “desperate urge to breathe.” You may have experienced this sensation at the end of a prolonged breath-hold, and it is the unpleasantness of air hunger that made you resume breathing. “Air hunger” is a warning signal that ventilation is insufficient and blood gases are becoming deranged; given the immediate importance of maintaining constant blood gases, air hunger is perhaps our most important homeostatic signal, and it has been referred to as the “suffocation alarm.” The mechanisms underlying air hunger are still unclear, but again, they were once thought to involve the respiratory muscle motor and sensory signals and detection of a disparity between them—that is, the brain perceived that the respiratory muscles were not achieving the work they had been commanded to do. This hypothesis was developed in the sixties and still persists in texts today; however, it is wrong. In two separate labs, one at Harvard University and the other in Australia, pulmonary physiologists completely paralyzed each other to remove all motor activity; when they inhaled carbon dioxide, they still felt air hungry, suggesting the respiratory muscle signals were not essential to generate air hunger. So where does air hunger come from?
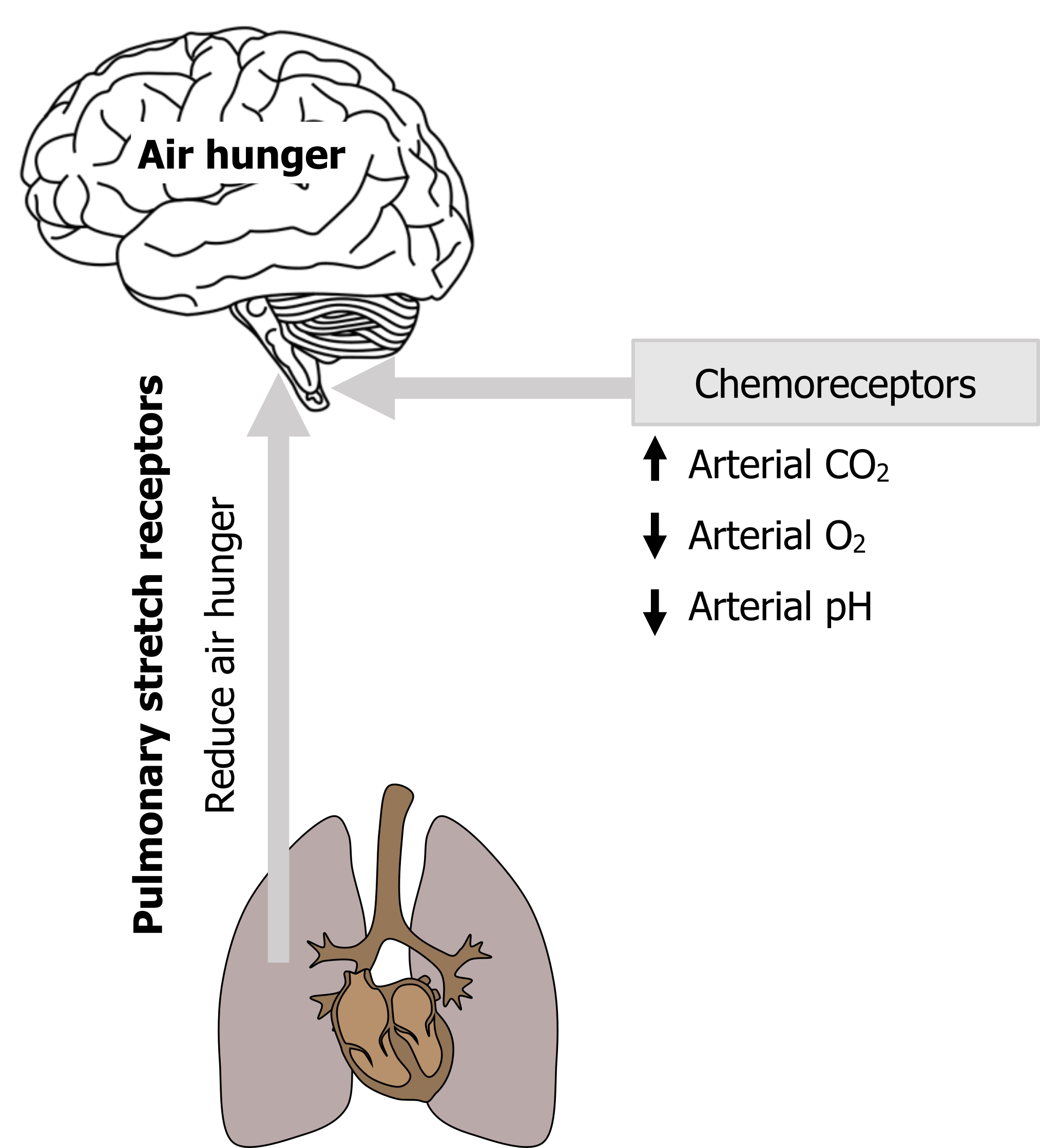
We see air hunger arise when PaCO2 rises, when PaO2 falls, or when arterial pH decreases. These changes are detected by chemoreceptors that reflexly increase the drive to breathe from the brainstem. While we are not usually aware of our reflex breathing drive, we think that once this drive increases to a critical level, a signal is sent upward that is perceived as air hunger.
So any signals to the brainstem respiratory networks that increase the drive to breathe are likely to promote air hunger, and these influences may not all be chemical (see figure 18.3). For example, emotions such as anxiety increase the drive to breathe, and this is a pertinent point with clinical ramifications that we will return to.
Likewise, any influences that reduce the drive to breathe also have a tendency to reduce air hunger (see figure 18.3). Perhaps the most interesting example of this is the effect of pulmonary stretch receptor activity. Pulmonary stretch receptors are mechanoreceptors in the airways that respond to lung inflation. Although this pulmonary afferent activity is thought to have little effect on the control of breathing in man, it reduces the drive to breathe in other species as part of the Hering–Breuer reflex. What we see in humans is that lung inflation, and presumably an increase in pulmonary stretch receptor firing, profoundly reduces air hunger, even in the absence of any blood gas improvements.
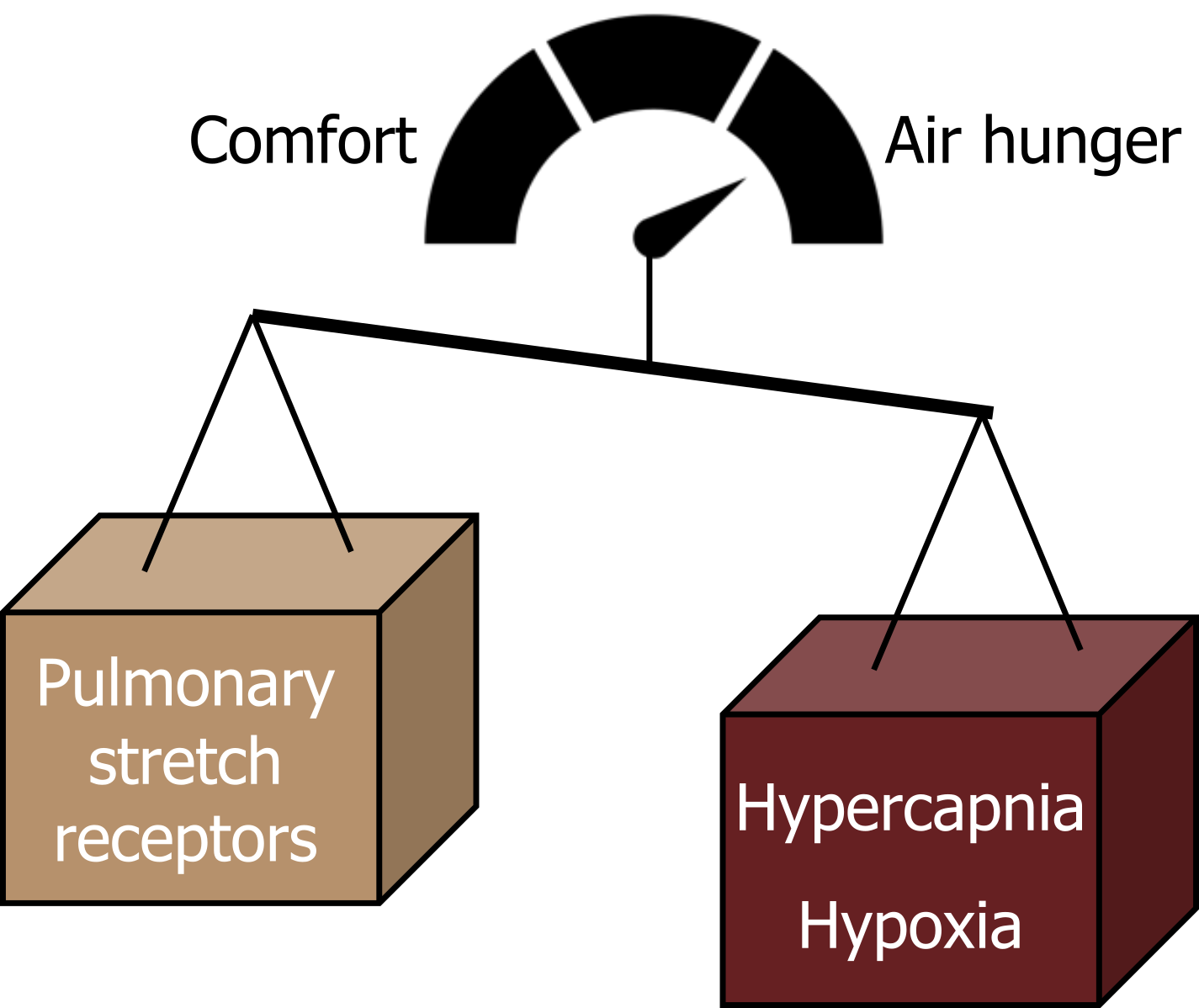
This is easy to demonstrate to yourself by holding your breath; during the breath-hold CO2 will gradually accumulate in your bloodstream and you will feel a gradually increasing urge to breathe that will become increasingly more uncomfortable to a point when it is intolerable and you must begin breathing again. That first big breath you take does not return your arterial CO2 to normal, but despite this you get great relief from air hunger by taking it, probably because that big breath stretched the lung and caused a rapid increase of stretch receptor activity to the brainstem.
So air hunger is really affected by a balance of influences: those that increase the drive to breathe (such as hypercapnia and hypoxia) promote air hunger, while inhibitory influences on the drive to breathe tend to promote comfort (see figure 18.4).
Impact of Dyspnea
There are other elements that should be considered when dealing with the air-hungry patient. Probably because of its homeostatic importance, the sensation of air hunger is very effective at getting attention and producing fear and anxiety. Recent comparisons of attentive and emotional impacts suggest air hunger is perceived as much more threatening and worrisome than pain at equivalent intensities. (Ironically we routinely ask about patients’ pain, but rarely about their air hunger.)
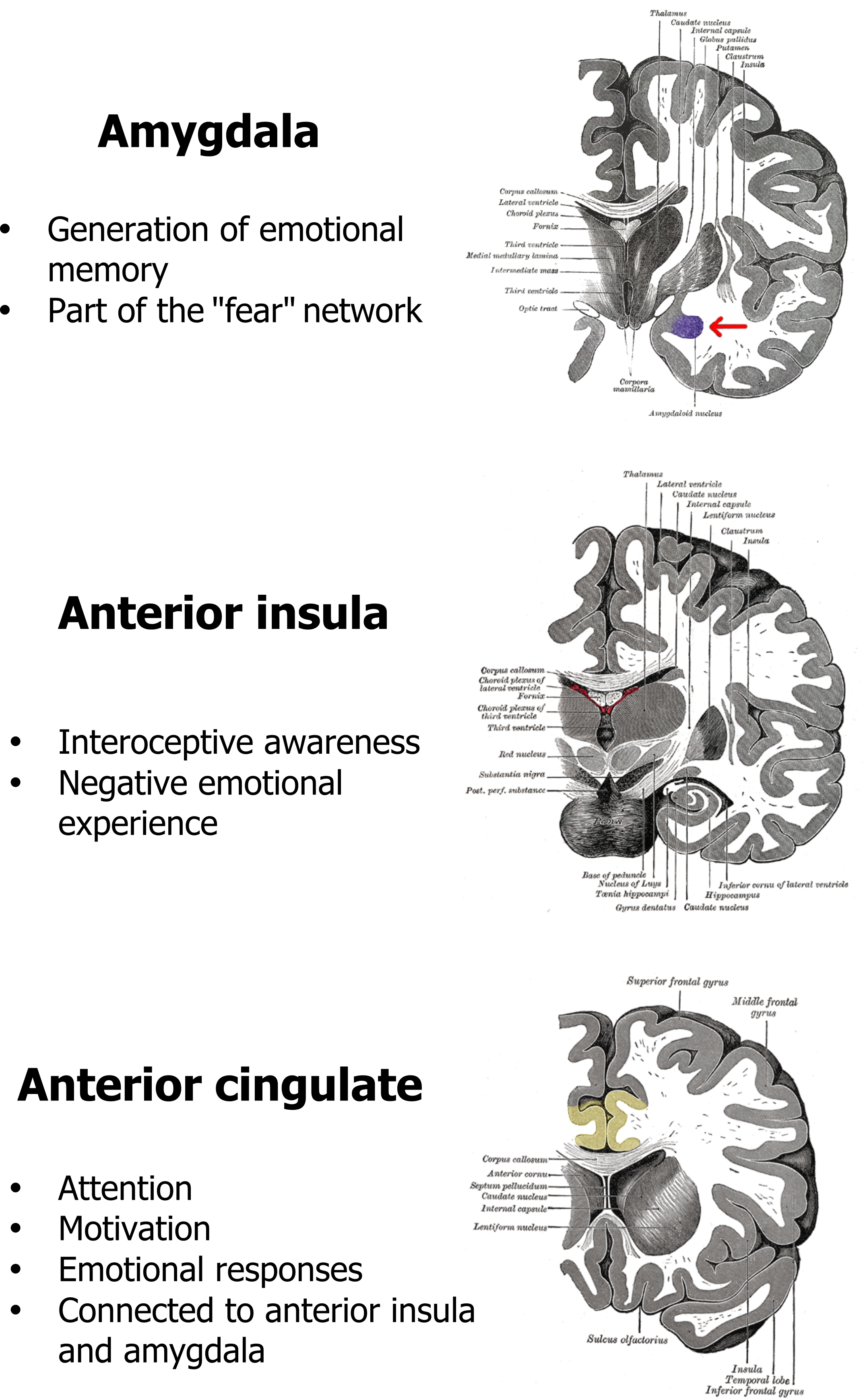
This emotional impact of air hunger is reflected in the regions of the brain that are consistently seen to be activated in recent functional brain imaging studies (see figure 18.5). The amygdala, anterior insula, and anterior cingulate are all persistently seen to activate during air hunger, and all are either associated with the brain’s fear network or generation of emotional responses. The activation of the anterior insula is also interesting as this phylogenically old part of the cortex also responds to other homeostatic imbalances, such as thirst, hunger for food, and pain. Although air hunger itself is unpleasant, it is these emotional components that produce air hunger’s profoundly negative effect on patients’ quality of life and makes end-of-life distressing for both the patient and their loved ones. We will come back to the impact of emotional responses in a moment.
So there we are—three different forms of dyspnea, with separate neural mechanisms. That said, it is unlikely that a patient will ever walk into your office and tell you they have “dyspnea,” or pinpoint which form of dyspnea they have. But taking an interest in the subtleties of your patients’ comments may not be a purely academic exercise either. More likely they are likely to use descriptors like those shown in table 18.1. These descriptors that use more common, everyday language have been related to each form of dyspnea. Knowing which form or forms of dyspnea the patient is experiencing can help in diagnosis as the different causes of dyspnea (like those listed in figure 18.1) can produce different levels of each form. For example, chest tightness is much more commonly reported by asthmatics, whereas patients with chronic obstructive pulmonary disease tend to use descriptors more related to effort to breathe and air hunger.
Form of dyspnea | Common descriptors |
---|---|
Air hunger | - I feel that I am suffocating - I feel out of breath - I cannot get enough air - I am gasping for air - My breath does not go in all the way - I feel a hunger for more air |
Effort to breathe | - I feel that my breathing is rapid - I feel that I am breathing more - My breathing requires effort - My breathing is heavy - My breathing is shallow |
Chest tightness | - My chest feels tight - My chest is constricted |
Table 18.1: Patient descriptors for the three different forms of dyspnea.
While the different forms of dyspnea have been investigated, described, and now explored as their potential as diagnostic tools, the emotional impact of dyspnea is only now receiving more attention. The most immediate complication caused by the emotional component is the potential for a positive feedback loop to form between air hunger and the anxiety it generates. The anxiety that air hunger produces results in an increased drive to breathe; in turn this increased drive to breathe causes the air hunger to increase, which leads to more anxiety and so on (figure 18.6).
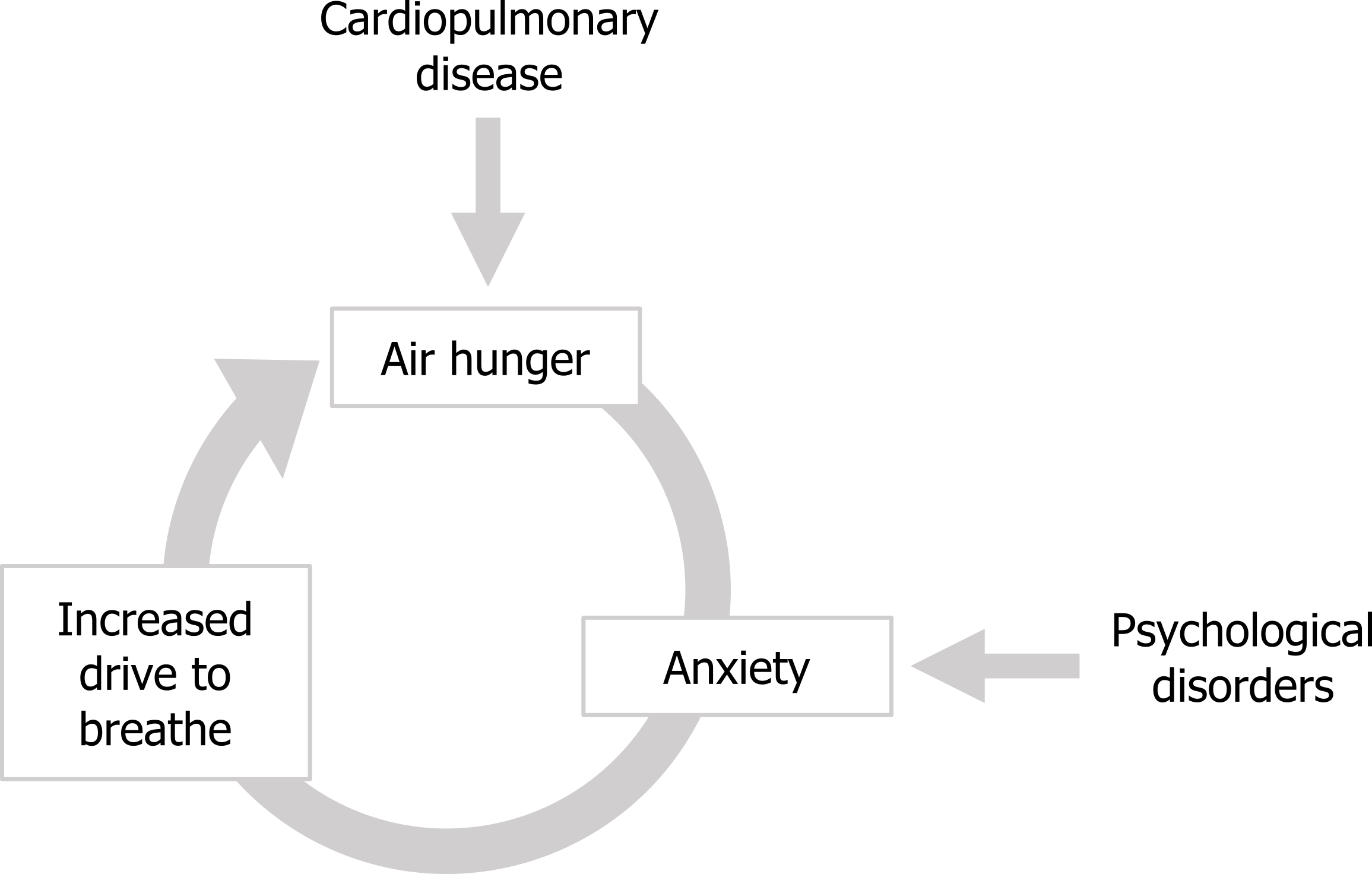
Behavioral effects of dyspnea: This cycle can be entered into by different types of patients; those with cardiopulmonary disease enter the cycle at the point of the air hunger, whereas patients with anxiety disorders can enter the cycle at this point and can experience significant air hunger even with apparently perfectly normal lung and heart function. On a more long-term basis the quality of life of air-hungry patients can be diminished by another positive feedback scenario that can produce “respiratory cripples” of cardiopulmonary patients. The air hunger produced by the underlying disease worsens during exertion, so makes exercising uncomfortable. This frequently results in patients avoiding exercise, perhaps starting with taking an elevator instead of the stairs, or driving to the grocery store when previously they might have walked.
This reduction in exercise leads to cardiac deconditioning, which in turn makes the air hunger worse and leads to further avoidance of exercise. Along with the progression of the disease, this cycle may leave the patient out-of-breath while simply sitting in a chair.
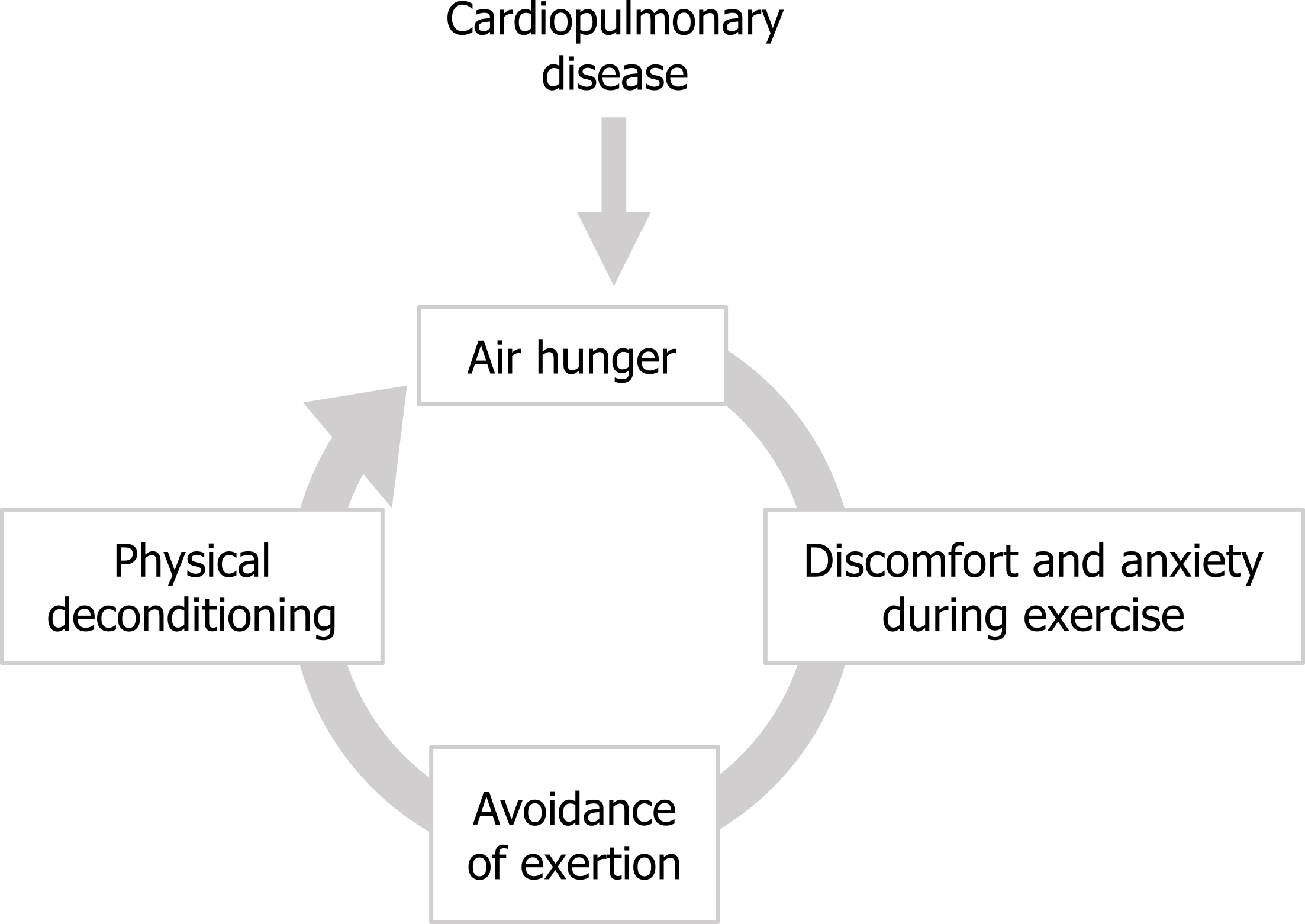
The patient’s quality of life becomes severely diminished as their life is ruled by dyspnea that prevents them from leaving the house, interacting with children or grandchildren, and performing simple activities that used to bring enjoyment, such as gardening, wood-working, walking, and more. This reduced quality of life can potentially lead to depression, and the emotional response to dyspnea may be exacerbated.
So what can be done to relieve the patient’s air hunger and the associated anxiety? Well, despite its prevalence, the treatment of dyspnea is decades behind the treatment of pain. For too long the approach to treating dyspnea has been to treat the underlying disease with the expectation that the dyspnea will go away. This is true and a perfect course of action for many conditions, but for many diseases that produce dyspnea we have ineffective cures, such as emphysema, lung cancer, and pulmonary fibrosis. How do we make the 49 percent of terminally ill patients who suffer with dyspnea at the end of life more comfortable?
Opioids: A common practice is to use opioids, but the mechanism of how they might work and indeed their overall efficacy has been disputed. There are a number of routes for how morphine may act, if it indeed does so. Opioids may have a direct inhibitory effect on the central networks that generate air hunger, or at higher dose concentrations they may reduce air hunger indirectly by causing respiratory depression—that is, they tackle air hunger at what we think to be its source. Alternatively, opioids may reduce the affective or emotional component of dyspnea (i.e., the patient may perceive air hunger, but simply is not as bothered by it). Recent work from Harvard University suggests that morphine has a direct effect on both the sensory and affective components of air hunger independent of its effect on ventilatory drive.
Anxiolytics: As the emotional component of air hunger is so strong, the fear and anxiety produced can be treated in the absence of any specific drug to treat the air hunger itself. Use of anxiolytics drugs has also produced mixed results that may be complicated by the patient’s underlying condition, and whether the type of anxiolytic causes ventilatory depression.
Furosemide: Although there is currently no drug that specifically tackles air hunger, there is a growing body of evidence that inhaled furosemide (the loop diuretic) reduces air hunger by sensitizing pulmonary stretch receptors, meaning they fire more for any given lung volume. This amplifies the stretch receptors’ inhibitory effect on air hunger described earlier, by fooling the brain into thinking the lungs are at a greater volume than they really are.
Nonpharmaceutical alternatives: A nonpharmaceutical alternative is to simply cool the patient’s face with a fan or wet cloth. This facial cooling initiates the “diving reflex” via the trigeminal nerve. One component of the diving reflex is to reduce ventilatory drive at the brainstem—an ideal response if one is heading underwater.
This inhibition of ventilatory drive is likely responsible for the moderate reduction in air hunger seen with facial cooling.
Rehabilitation and desensitization: While dyspnea can be addressed by other methods than those briefly described here, few have been shown to work consistently or effectively. On a more long-term basis, breathing training and pulmonary rehabilitation appear to help patients overcome exacerbations of their disease or even reduce chronic air hunger, but both require patient cooperation and compliance and may have limited effect in severe disease. What can be taken from the literature is that the treatment of dyspnea is in desperate need of more attention. For a symptom that is so common and has such an impact on patients, dyspnea is a clinical issue that is woefully underaddressed.
References, Resources, and Further Reading
Text
American Thoracic Society Committee on Dyspnea. “An Official American Thoracic Society Statement: Update on the Mechanisms, Assessment, and Management of Dyspnea.” American Journal of Respiratory and Critical Care Medicine 185, no. 4: 435–52. https://doi.org/10.1164/rccm.201111-2042ST.
Banzett, Robert B., Robert W. Lansing, and Andrew P. Binks. “Air Hunger: A Primal Sensation and a Primary Element of Dyspnea.” Comprehensive Physiology 11, no. 2 (April 2021): 1449–83. https://doi.org/10.1002/cphy.c200001.
Figures
Figure 18.1: Conditions that can produce dyspnea. Grey, Kindred. 2022. CC BY 4.0. https://archive.org/details/18.1_20220125
Figure 18.2: Types of dyspnea. Grey, Kindred. 2022. CC BY 4.0. https://archive.org/details/18.2_20220125
Figure 18.3: The proposed neural mechanism of air hunger. Grey, Kindred. 2022. CC BY 4.0. Added Brain by Clockwise from Noun Project. CC BY 3.0. https://archive.org/details/18.3_20220125
Figure 18.4: Balance of pulmonary stretch receptor and chemoreceptor firing. Grey, Kindred. 2022. CC BY 4.0. Added credit score by akash k from Noun Project. CC BY 3.0. https://archive.org/details/18.4_20220125
Figure 18.5: Central regions associated with air hunger. Grey, Kindred. 2022. CC BY 4.0. Added Gray 718-amygdala by Henry Vandyke Carter from WikimediaCommons. Public domain. Added Gray717 by Henry Vandyke Carter from WikimediaCommons. Public domain. Added Gray743 cingulate gyrus by Henry Vandyke Carter (colored by was_a_bee) from WikimediaCommons. Public domain. https://archive.org/details/
Figure 18.6: The cycle of anxiety causing an increase in the drive to breathe and air hunger. Grey, Kindred. 2022. CC BY 4.0. https://archive.org/details/18.6_20220125/mode/1up
Figure 18.7: The cycle of worsening air hunger leading to exercise avoidance and physical deconditioning. Grey, Kindred. 2022. CC BY 4.0. https://archive.org/details/18.7_20220125/mode/1up