1 Fundamentals
Learning objectives
- Relate the anatomy of the airways, airspaces and cellular structure of the lungs to lung function.
The Components of Lung Function
The primary function of the pulmonary system is to maintain arterial blood gas homeostasis by gaining oxygen from the atmosphere and expelling carbon dioxide from the venous blood. Although initially appearing simple, this process is a summation of distinct components that are important to understand if one is to understand the mechanisms and management of pulmonary diseases. Each of these components are shown in the schematic of figure 1.1 and are addressed chapter by chapter in this book.
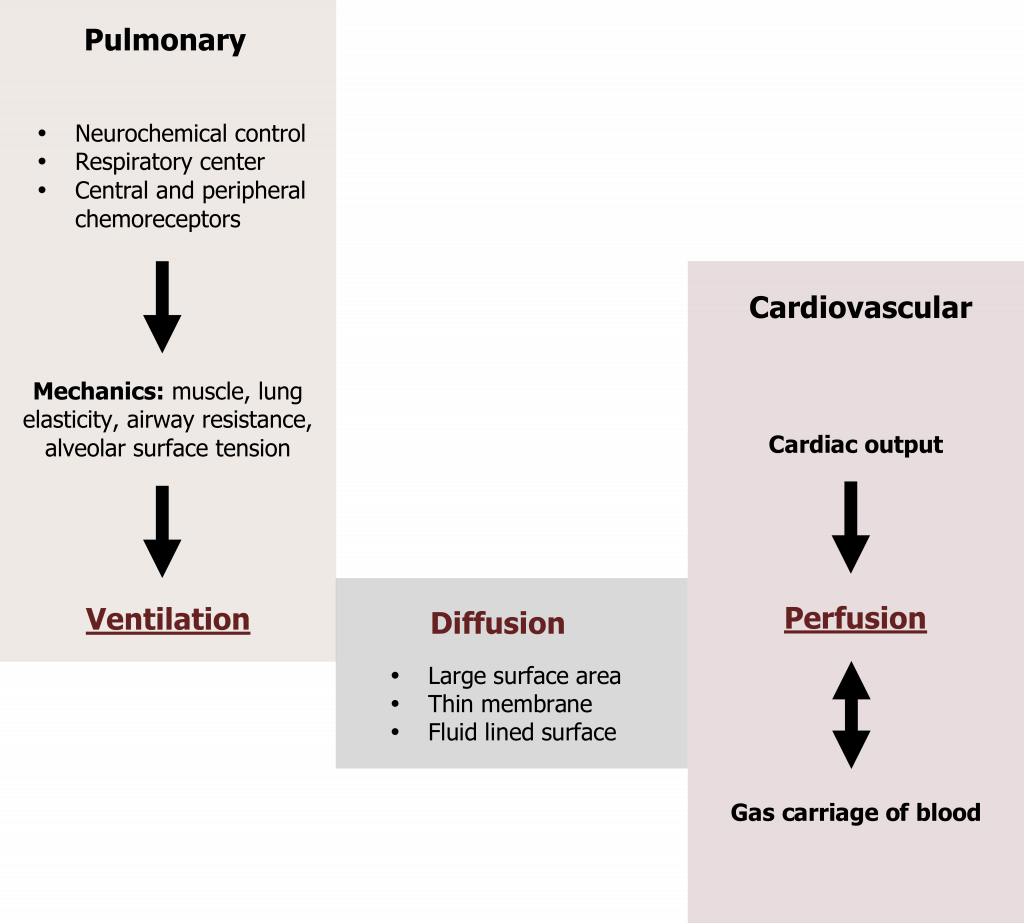
We will see how blood gases are monitored and maintained through neurochemical control of lung expansion and relaxation to achieve the appropriate level of alveolar ventilation. Factors that affect the degree of gas exchange between the lung and blood will be discussed, along with the coordination of ventilation and perfusion of the lung. Finally, we will see how oxygen and carbon dioxide are transported in the bloodstream to and from tissue and the mechanisms that ensure appropriate delivery and a stable blood gas environment. Before we begin, however, we will look at the functional anatomy of the lung and how the lung is well designed to perform its primary role and defend itself from the external environment.
Defense of the Lung
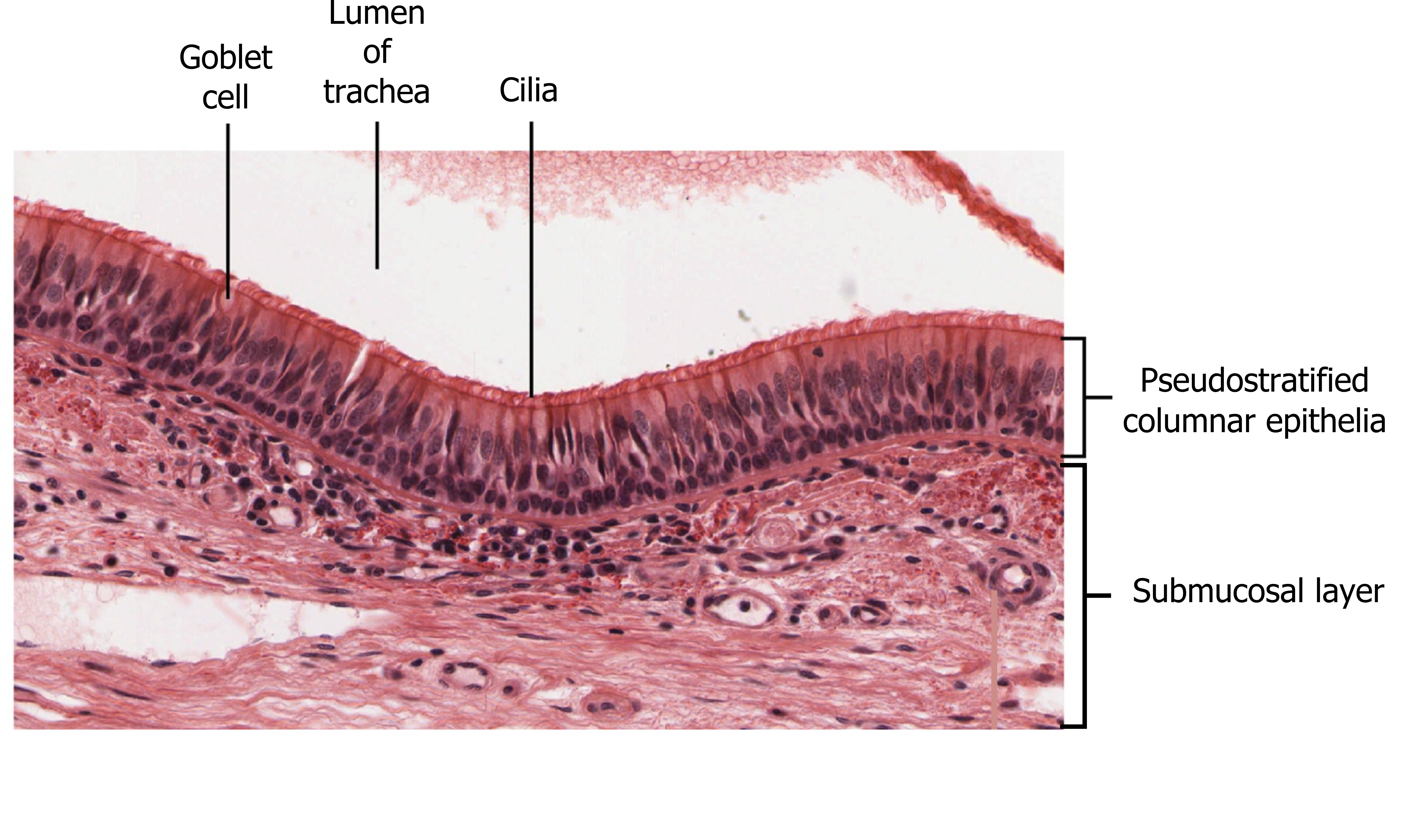
As the only internal organ exposed to the external environment, the lung needs special protection from particles or pathogens that could be transported down the airways with inhaled air. The first line of defense is the nasal cavity, which is lined with a ciliated epithelial, dispersed within which are goblet cells producing mucus (figure 1.2). This mucus forms a sticky layer on top of the epithelial surface and traps inhaled particles, bacteria, or other potential pathogens. The mucus is then moved by the cilia back toward the pharynx where it can be coughed or spat out.
The inhaled air also must be warmed and humidified before it reaches the gas exchange surfaces, otherwise the relatively cold and dry air would cause evaporation of the thin water layer lining the gas exchange surfaces that is essential for allowing gases to dissolve and diffuse into or out of the pulmonary bloodstream. This warming and humidification is achieved by transfer of heat and water from blood in the highly vascularized nasal cavity (figure 1.3).
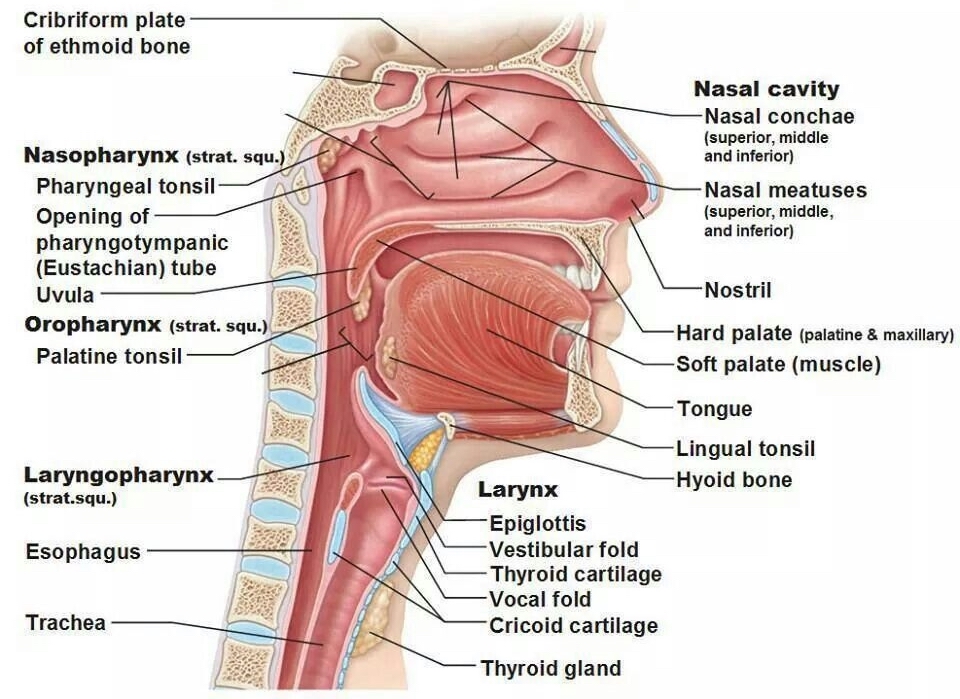
The second line of defense (which becomes more important when breathing through the mouth) is the lining of the trachea. Again, this is covered with a ciliated epithelium with mucus-producing goblet cells (figure 1.2). As in the nasal cavity, particles and potential pathogens are trapped in the mucus layer and cilia move the mucus up toward the mouth for expulsion. The trachea and larynx also contain sensory nerve endings (rapidly adapting receptors, nicknamed “irritant receptors”) that respond to the arrival of particles on the epithelial surface and initiate the cough reflex and propel the offending particles out of the airway (see more in chapter 17).
The Bronchial Tree
The airways, or bronchial tree (figure 1.4), consist of a series of branching tubes that become narrower and shorter but more numerous as they descend into the lung.
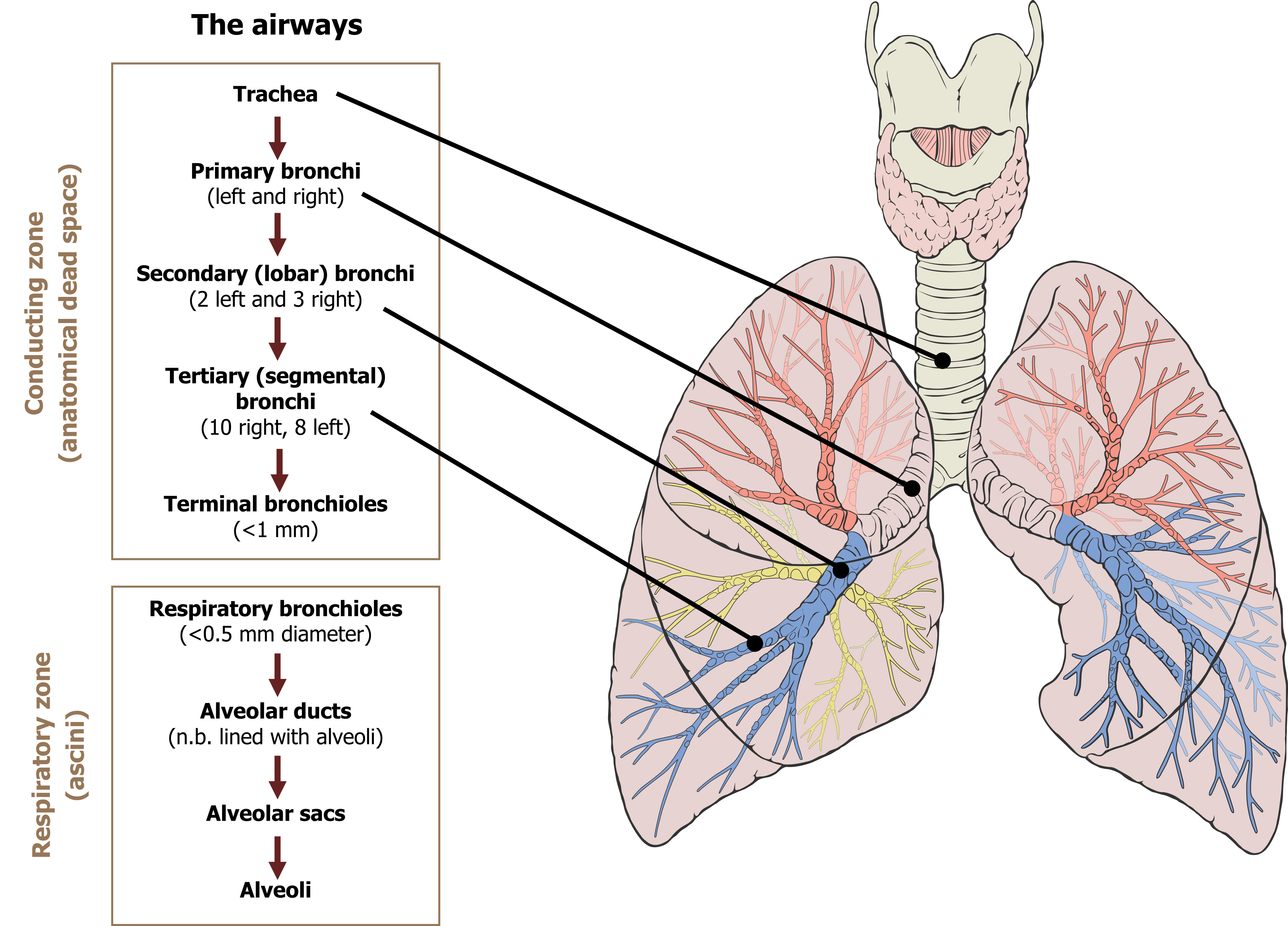
The trachea bifurcates into the primary bronchi, left and right, transporting air into the left and right lungs, respectively. The primary bronchi consequently divide into lobar (or secondary) bronchi, the number of which correspond to the number of lobes in each lung. The lobar bronchi then divide into segmental (or tertiary) bronchi to supply the segments of each lobe. This bifurcation process continues to the terminal bronchioles. This initial section of the bronchial tree is referred to as the conducting zone as its role is to transfer air to the gas exchange surfaces (figure 1.4). As no gas exchange takes place here, these airways constitute the anatomical dead space and have a volume of approximately 150 mL.
Each terminal bronchiole then divides into numerous respiratory bronchioles, the walls of which may contain some alveoli and are therefore capable of some gas exchange; this is the transition to the respiratory zone of the lung and the onset of gas exchange (figure 1.4). The respiratory zone becomes firmly established when terminal bronchioles divide into alveolar ducts that are fundamentally tubes lined with alveoli. These alveolar ducts then terminate in alveolar sacs. The portion of lung distal to each terminal bronchiole forms an anatomical unit called the acinus. Although only a few millimeters long, collectively these acini make up the respiratory zone and form the vast majority of the lung’s volume.
Flow in the Airways
Airflow down the bronchial tree is caused by the generation of a pressure differential when lung volume is expanded by contraction of the respiratory muscles (more on this in chapter 2). Inspired air first enters the airways of the conducting zone, which while having the largest diameter airways also has the fewest; consequently the total cross-sectional area of the conducting zone is relatively low. With a large volume of air passing through a low cross-sectional area, the velocity of air in the conducting zone is high and is moving by “bulk flow” generated by the pressure differential (like water through a hose) until it reaches the terminal bronchioles and the end of the conducting zone.
When this air enters the respiratory zone, it slows rapidly. This is due to the enormous total cross-sectional area of the airways in the respiratory zone—while the airways are much narrower here, they are far more numerous. The final transfer of the gases through the respiratory zone is therefore achieved by diffusion; the rate of diffusion is so rapid and the distances so short that concentration differences are abolished within a second.
As an aside, this deceleration of the air at the terminal bronchioles means any particles that have been able to descend this deep are frequently deposited here. This has ramifications for disease and also delivery of inhaled medications.
Primary Objective: Gas Exchange
Gases that have diffused to the respiratory zone find themselves in an ideal environment for gas exchange. We will deal with gas exchange in more detail later (chapter 7), but you should appreciate that the essential components for efficient gas exchange are all present in the lung.
A large surface area is generated by the 500 million alveoli, and while each alveolus only has a diameter of 0.3 mm, collectively they produce a total gas exchange surface of 100 m2—about the surface area of a tennis court.
The membranes that gases transfer across are very thin and pose little opposition. In brief, oxygen entering the alveolus only has to cross the squamous cell of the alveolus wall, a very thin basement membrane, and then the squamous cell of the capillary wall to get into the pulmonary circulation. The total distance can be as low as 0.2 micrometers. This degree of thinness also makes these membranes prone to damage.
As well as the alveolus receiving air (that is being ventilated), the other essential component for gas exchange is blood flow. This is provided by the pulmonary circulation and consists of all cardiac output coming from the right heart. The pulmonary circulation forms very dense networks of capillaries surrounding each alveolus, so much so that the alveoli can be imagined as being washed over with blood.
These characteristics make the lung a highly efficient exchange organ between the environment and the circulation, which is ideal for the transfer of O2 into the bloodstream and, just as importantly, CO2 out. It also allows some drugs to be delivered by inhalation, but also has the potential to allow noxious substances into the bloodstream. Likewise, changes in any of these characteristics of the lung in disease, such as loss of surface area in emphysema or membrane thickening in pulmonary fibrosis, can severely diminish gas exchange.
References, Resources, and Further Reading
Text
Levitsky, Michael G. “Chapter 1: Function and Structure of the Respiratory System.” In Pulmonary Physiology, 9th ed. New York: McGraw Hill Education, 2018.
West, John B. “Chapter 1: Structure and Function—How the Architecture of the Lung Subserves Its Function.” In Respiratory Physiology: The Essentials, 9th ed. Philadelphia: Wolters Kluwer Health/Lippincott Williams and Wilkins, 2012.
Widdicombe, John G., and Andrew S. Davis. “Chapter 1.” In Respiratory Physiology. Baltimore: University Park Press, 1983.
Figures
Figure 1.1: Components of gas exchange. Grey, Kindred. 2022. CC BY 4.0. https://archive.org/details/1.1_20220125/mode/1up
Figure 1.2: The mucociliary escalator. OpenStax College. 2013. CC BY 3.0. https://commons.wikimedia.org/wiki/File:2304_Pseudostratified_Epithelium.jpg
Figure 1.3: Air conditioning. Chukquemeka, Uchechukwu. 2018. “Free to be used and shared.” https://www.physio-pedia.com/File:Upper_respiratory_system_2.jpg#filelinks
Figure 1.4: The bronchial tree. Grey, Kindred. 2022. CC BY 4.0. Added Lung by Lynch, Patrick J. from WikimediaCommons. https://archive.org/details/1.5._20220125/mode/1up