6 Metamorphic Rocks
Contributing Author: Dr. Peter Davis, Pacific Lutheran University
Learning Objectives
By the end of this chapter, students will be able to:
- Describe the temperature and pressure conditions of the metamorphic environment.
- Identify and describe the three principal metamorphic agents.
- Describe what recrystallization is and how it affects mineral crystals.
- Explain what foliation is and how it results from directed pressure and recrystallization.
- Explain the relationships among slate, phyllite, schist, and gneiss in terms of metamorphic grade.
- Define index mineral.
- Explain how metamorphic facies relate to plate tectonic processes.
- Describe what a contact aureole is and how contact metamorphism affects surrounding rock.
- Describe the role of hydrothermal metamorphism in forming mineral deposits and ore bodies.
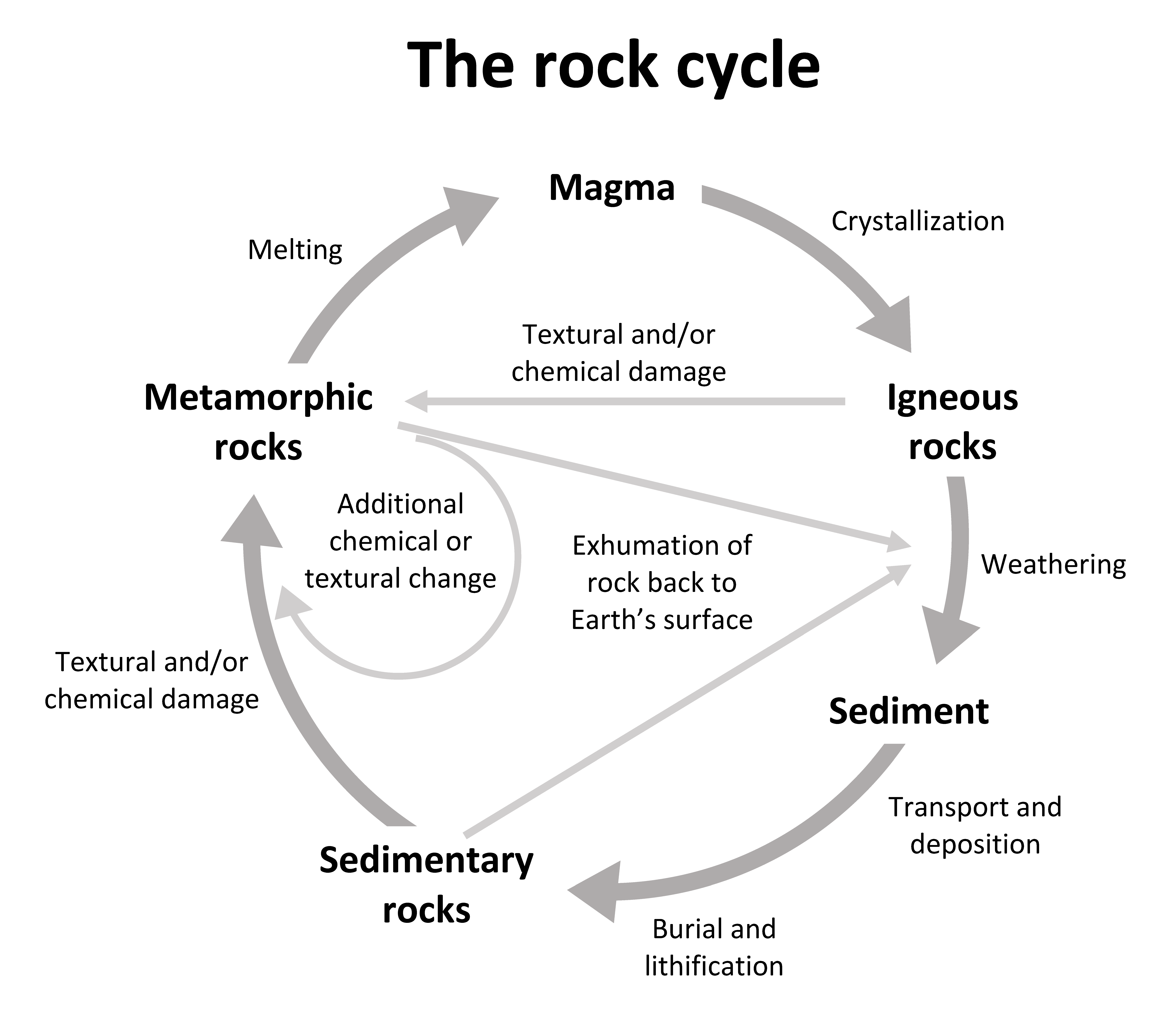
Metamorphic rocks, meta- meaning change and –morphos meaning form, is one of the three rock categories in the rock cycle (see chapter 1). Metamorphic rock material has been changed by temperature, pressure, and/or fluids. The rock cycle shows that both igneous and sedimentary rocks can become metamorphic rocks. And metamorphic rocks themselves can be re-metamorphosed. Because metamorphism is caused by plate tectonic motion, metamorphic rock provides geologists with a history book of how past tectonic processes shaped our planet.
6.1 Metamorphic Processes
Metamorphism occurs when solid rock changes in composition and/or texture without the mineral crystals melting, which is how igneous rock is generated. Metamorphic source rocks, the rocks that experience the metamorphism, are called the parent rock or protolith, from proto– meaning first, and lithos- meaning rock. Most metamorphic processes take place deep underground, inside the earth’s crust. During metamorphism, protolith chemistry is mildly changed by increased temperature (heat), a type of pressure called confining pressure, and/or chemically reactive fluids. Rock texture is changed by heat, confining pressure, and a type of pressure called directed stress.
6.1.1 Temperature (Heat)
Temperature measures a substance’s energy—an increase in temperature represents an increase in energy. Temperature changes affect the chemical equilibrium or cation balance in minerals. At high temperatures atoms may vibrate so vigorously they jump from one position to another within the crystal lattice, which remains intact. In other words, this atom swapping can happen while the rock is still solid.
The temperatures of metamorphic rock lies in between surficial processes (as in sedimentary rock) and magma in the rock cycle. Heat-driven metamorphism begins at temperatures as cold as 200˚C, and can continue to occur at temperatures as high as 700°C-1,100°C. Higher temperatures would create magma, and thus, would no longer be a metamorphic process. Temperature increases with increasing depth in the Earth along a geothermal gradient (see chapter 4) and metamorphic rock records these depth-related temperature changes.
6.1.2 Pressure
Pressure is the force exerted over a unit area on a material. Like heat, pressure can affect the chemical equilibrium of minerals in a rock. The pressure that affects metamorphic rocks can be grouped into confining pressure and directed stress. Stress is a scientific term indicating a force. Strain is the result of this stress, including metamorphic changes within minerals.
Confining Pressure
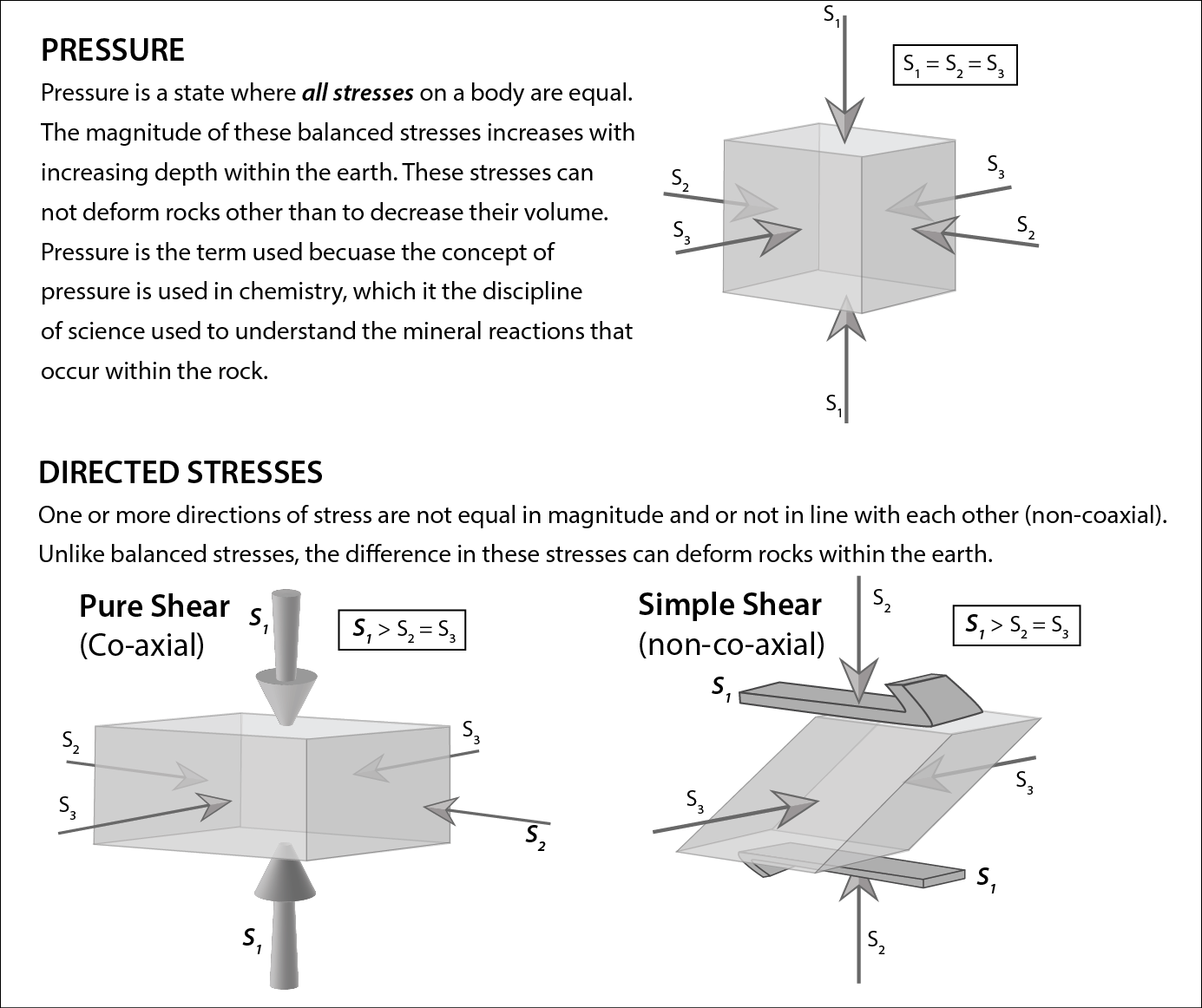
Pressure exerted on rocks under the surface is due to the simple fact that rocks lie on top of one another. When pressure is exerted from rocks above, it is balanced from below and sides, and is called confining or lithostatic pressure. Confining pressure has equal pressure on all sides (see figure 6.2) and is responsible for causing chemical reactions to occur just like heat. These chemical reactions will cause new minerals to form.
Confining pressure is measured in bars and ranges from 1 bar at sea level to around 10,000 bars at the base of the crust. For metamorphic rocks, pressures range from a relatively low-pressure of 3,000 bars around 50,000 bars, which occurs around 15-35 kilometers below the surface.
Directed Stress
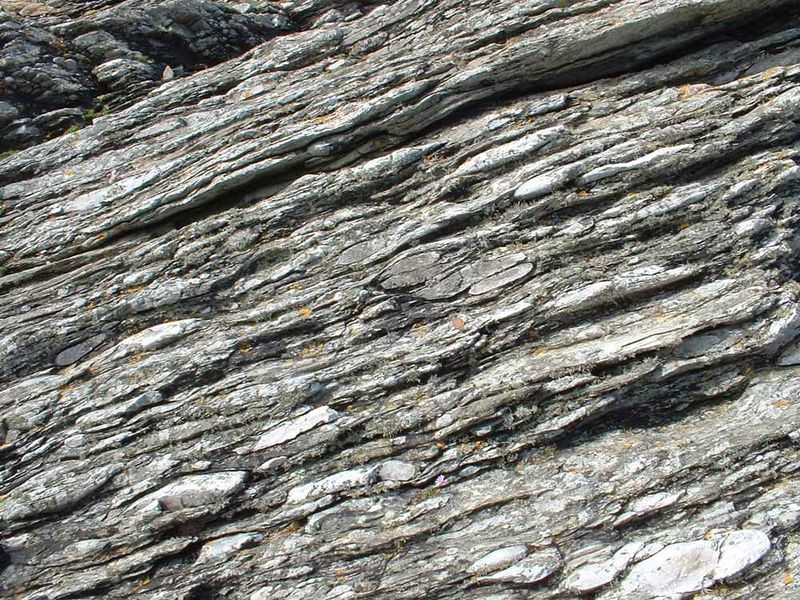
Directed stress, also called differential or tectonic stress, is an unequal balance of forces on a rock in one or more directions (see previous figure). Directed stresses are generated by the movement of lithospheric plates. Stress indicates a type of force acting on rock. Strain describes the resultant processes caused by stress and includes metamorphic changes in the minerals. In contrast to confining pressure, directed stress occurs at much lower pressures and does not generate chemical reactions that change mineral composition and atomic structure. Instead, directed stress modifies the parent rock at a mechanical level, changing the arrangement, size, and/or shape of the mineral crystals. These crystalline changes create identifying textures, which is shown in the figure below comparing the phaneritic texture of igneous granite with the foliated texture of metamorphic gneiss.
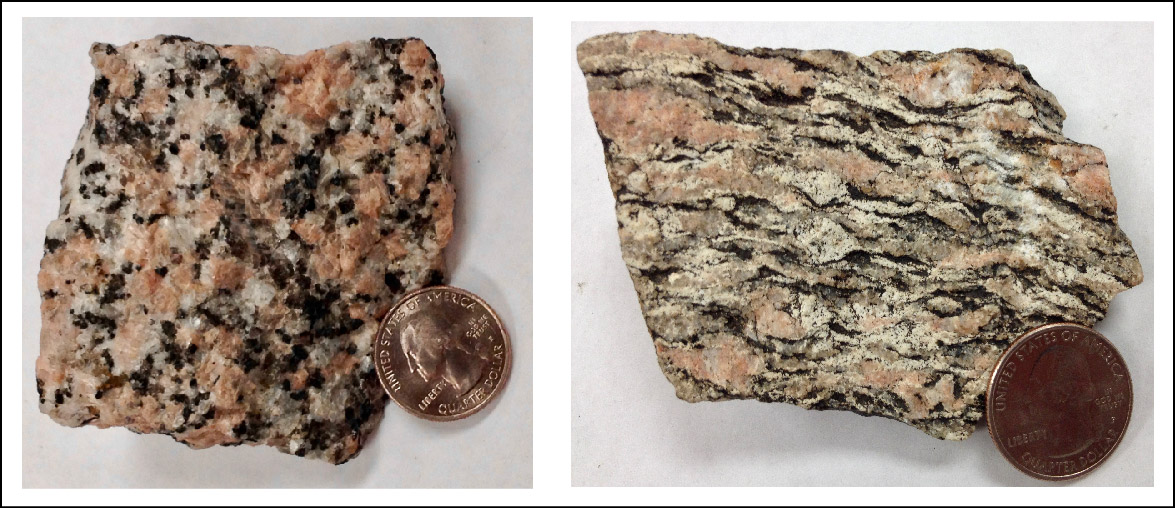
Directed stresses produce rock textures in many ways. Crystals are rotated, changing their orientation in space. Crystals can get fractured, reducing their grain size. Conversely, they may grow larger as atoms migrate. Crystal shapes also become deformed. These mechanical changes occur via recrystallization, which is when minerals dissolve from an area of rock experiencing high stress and precipitate or regrow in a location having lower stress. For example, recrystallization increases grain size much like adjacent soap bubbles coalesce to form larger ones. Recrystallization rearranges mineral crystals without fracturing the rock structure, deforming the rock like silly putty; these changes provide important clues to understanding the creation and movement of deep underground rock faults.
6.1.3 Fluids
A third metamorphic agent is chemically reactive fluids that are expelled by crystallizing magma and created by metamorphic reactions. These reactive fluids are made of mostly water (H2O) and carbon dioxide (CO2), and smaller amounts of potassium (K), sodium (Na), iron (Fe), magnesium (Mg), calcium (Ca), and aluminum (Al). These fluids react with minerals in the protolith, changing its chemical equilibrium and mineral composition, in a process similar to the reactions driven by heat and pressure. In addition to using elements found in the protolith, the chemical reaction may incorporate substances contributed by the fluids to create new minerals. In general, this style of metamorphism, in which fluids play an important role, is called hydrothermal metamorphism or hydrothermal alteration. Water actively participates in chemical reactions and allows extra mobility of the components in hydrothermal alteration.
Fluids-activated metamorphism is frequently involved in creating economically important mineral deposits that are located next to igneous intrusions or magma bodies. For example, the mining districts in the Cottonwood Canyons and Mineral Basin of northern Utah produce valuable ores such as argentite (silver sulfide), galena (lead sulfide), and chalcopyrite (copper iron sulfide), as well as the native element gold. These mineral deposits were created from the interaction between a granitic intrusion called the Little Cottonwood Stock and country rock consisting of mostly limestone and dolostone. Hot, circulating fluids expelled by the crystallizing granite reacted with and dissolved the surrounding limestone and dolostone, precipitating out new minerals created by the chemical reaction. Hydrothermal alternation of mafic mantle rock, such as olivine and basalt, creates the metamorphic rock serpentinite, a member of the serpentine subgroup of minerals. This metamorphic process happens at mid-ocean spreading centers where newly formed oceanic crust interacts with seawater.
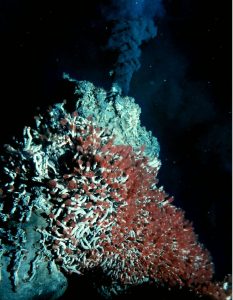
Some hydrothermal alterations remove elements from the parent rock rather than deposit them. This happens when seawater circulates down through fractures in the fresh, still-hot basalt, reacting with and removing mineral ions from it. The dissolved minerals are usually ions that do not fit snugly in the silicate crystal structure, such as copper. The mineral-laden water emerges from the sea floor via hydrothermal vents called black smokers, named after the dark-colored precipitates produced when the hot vent water meets cold seawater (see chapter 4). Ancient black smokers were an important source of copper ore for the inhabitants of Cyprus (Cypriots) as early as 4,000 BCE, and later by the Romans.
Take this quiz to check your comprehension of this section.
If you are using an offline version of this text, access the quiz for section 6.1 via the QR code.
6.2 Metamorphic Textures
Metamorphic texture is the description of the shape and orientation of mineral grains in a metamorphic rock. Metamorphic rock textures are foliated, non-foliated, or lineated are described below.
Identify rock's foliation | Textural features | Mineral composition | Rock name | Parent rock | |
---|---|---|---|---|---|
Foliated (layered texture) | Fine-grained or no visible grains | Flat, slaty cleavage is well developed. Dense, microscopic grains may exhibit slight sheen (or dull luster). Clanky sound when struck. Breaks into hard, flat sheets. | Fine, microscopic clay or mica | Slate | Shale |
Finely crystalline; micas hardly discernible, but impart a sheen or luster. Breaks along wavy surfaces. | Dark silicates and micas | Phyllite | Siltstone or shale | ||
Medium- to coarse-grained | Schistose texture. Foliation formed by alignment of visible crystals. Rock breaks along scaly foliation surfaces. Medium to fine-grained. Sparkling appearance. | Common minerals include chlorite, biotite, muscovite, garnet, and hornblende. Recognizable minerals used as part of rock name. Porphyroblasts common. | Mica schist | Siltstone or shale | |
Garnet schist | |||||
Gneissic banding. Coarse-grained. Foliation present as minerals arranged into alternating light and dark layers giving the rock a banded texture in side view. Crystalline texture. No cleavage. | Light-colored quartz and feldspar; dark ferromagnesian minerals | Gneiss | Shale or granitic rocks | ||
Non-foliated (no layered texture) | Fine-grained or no visible grains | Medium- to coarse-grained crystalline structure. | Crystals of amphibole (hornblende) in blade-like crystals | Amphibolite | Basalt, gabbro, or ultramafic igneous rocks |
Microcrystalline texture. Glassy black sheen. Conchoidal fracture. Low density. | Fine, tar-like, organic makeup | Anthracite coal | Coal | ||
Dense and dark-colored. Fine or microcrystalline texture. Very hard. Color can range from gray, gray-green to black. | Microscopic dark silicates | Hornfels | Many rock types | ||
Microcrystalline or no visible grains with smooth, wavy surfaces. May be dull or glossy. Usually shades of green. | Serpentine. May have fibrous asbestos visible. | Serpentinite | Ultramafic igneous rocks or peridotite | ||
Fine- to coarse-grained | Microcrystalline or no visible grains. Can be scratched with a fingernail. Shades of green, gray, brown, or white. Soapy feel. | Talc | Soapstone or talc schist | Ultramafic igneous rocks | |
Crystalline. Hard (scratches glass). Breaks across grains. Sandy or sugary texture. Color variable; can be white, pink, buff, brown, red, purple. | Quartz grains fused together. Grains will not rub off like sandstone. | Quartzite | Quartz sandstone | ||
Finely crystalline (resembling a sugar cube) to medium or coarse texture. Color variable; white, pink, gray, among others. Fossils in some varieties. | Calcite or dolomite crystals tightly fused together. Calcite effervesces with HCl; dolomite effervesces only when powdered. | Marble | Limestone or dolostone | ||
Texture of conglomerate, but breaks across clasts as easily as around them. Pebbles may be stretched (lineated) or cut by rock cleavage. | Granules or pebbles are commonly granitic or jasper, chert, quartz, or quartzite. | Meta-conglomerate | Conglomerate |
Table 6.1: Metamorphic rock identification table.
6.2.1 Foliation and Lineation
Foliation is a term used that describes minerals lined up in planes. Certain minerals, most notably the mica group, are mostly thin and planar by default. Foliated rocks typically appear as if the minerals are stacked like pages of a book, thus the use of the term ‘folia’, like a leaf. Other minerals, with hornblende being a good example, are longer in one direction, linear like a pencil or a needle, rather than a planar-shaped book. These linear objects can also be aligned within a rock. This is referred to as a lineation. Linear crystals, such as hornblende, tourmaline, or stretched quartz grains, can be arranged as part of a foliation, a lineation, or foliation/lineation together. If they lie on a plane with mica, but with no common or preferred direction, this is foliation. If the minerals line up and point in a common direction, but with no planar fabric, this is lineation. When minerals lie on a plane AND point in a common direction; this is both foliation and lineation.
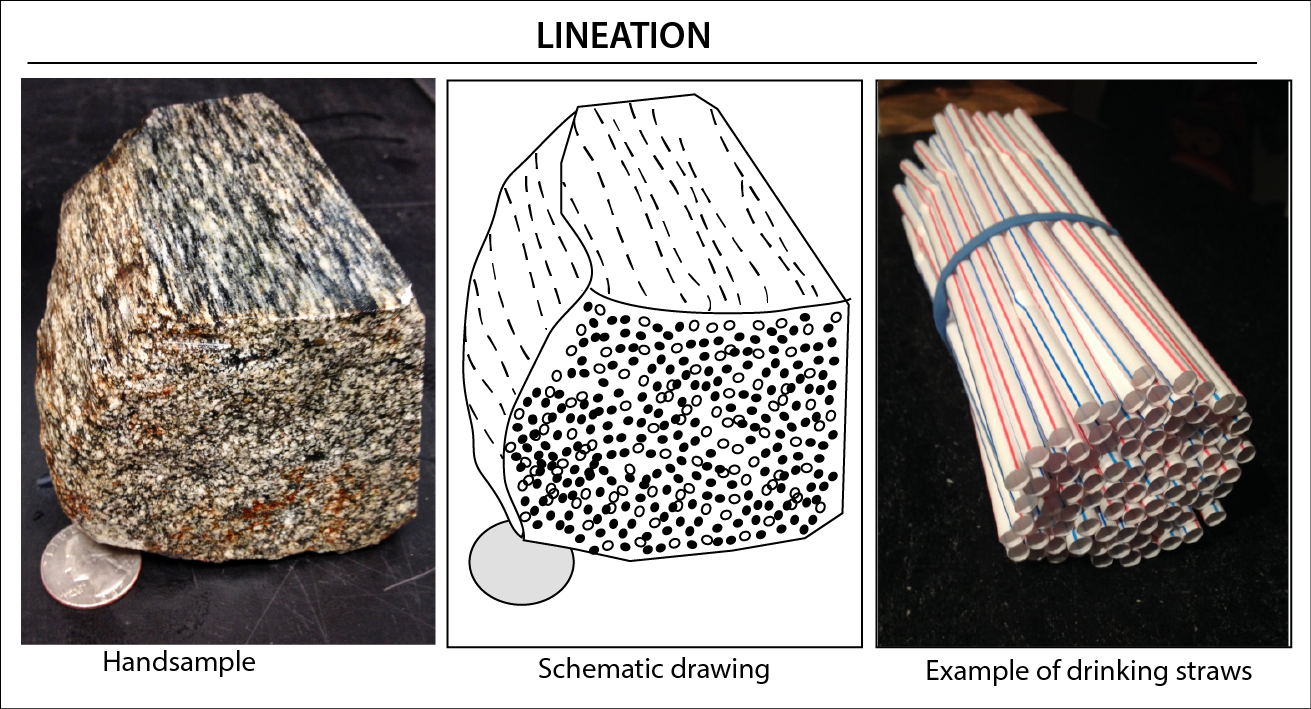
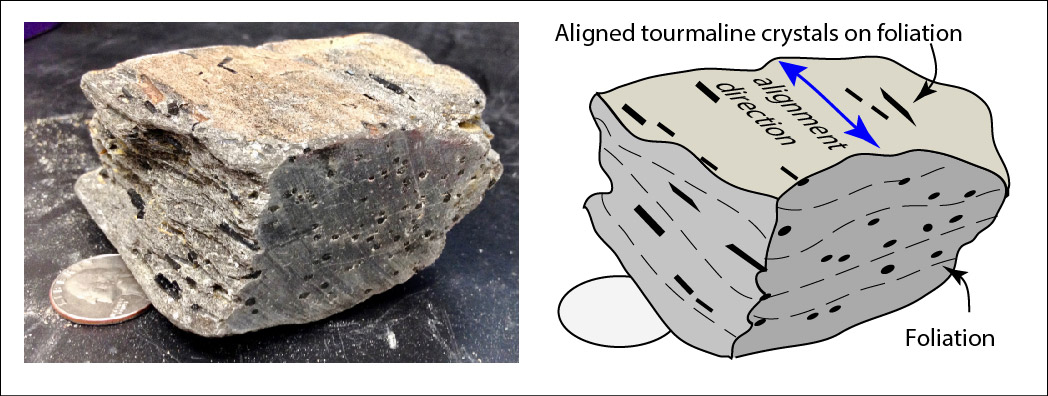
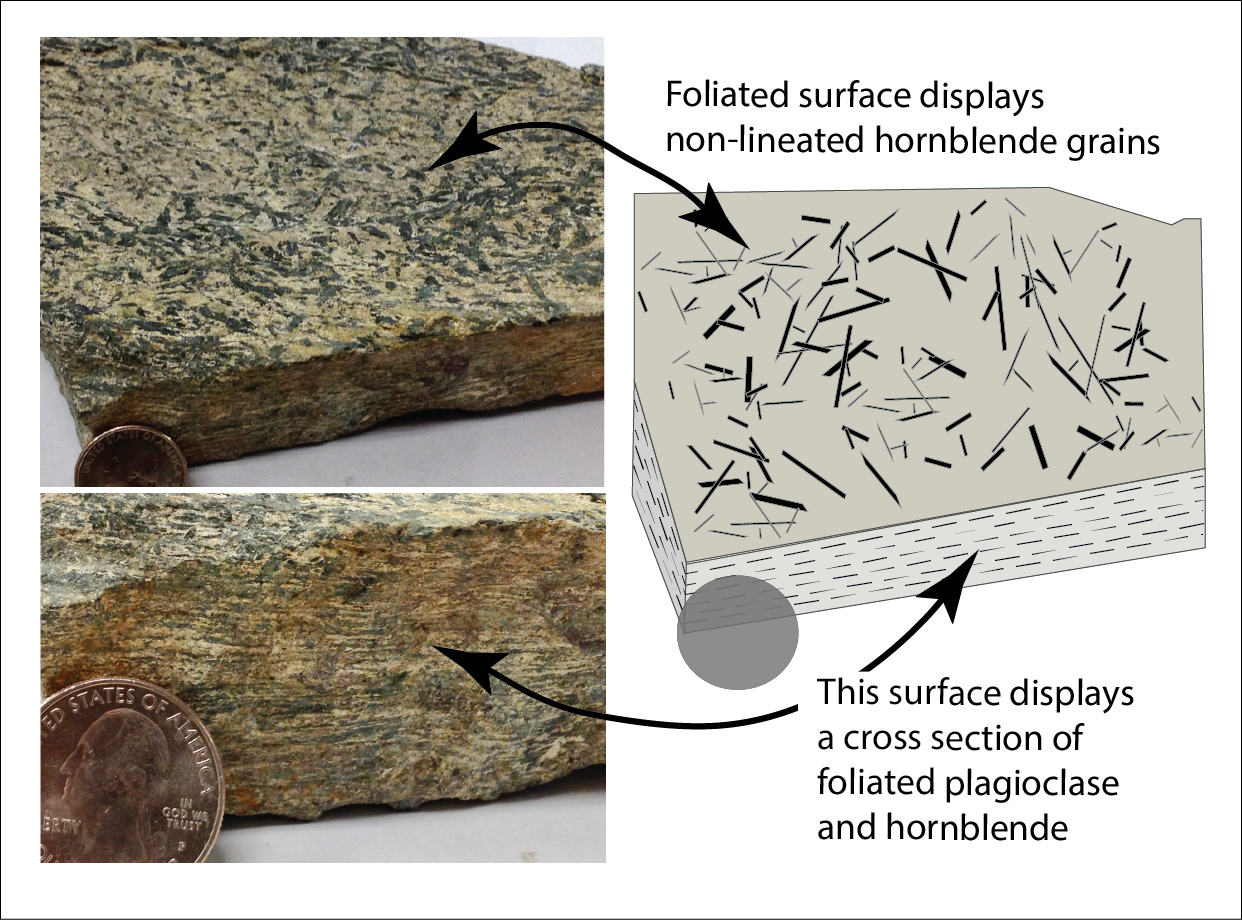
Foliated metamorphic rocks are named based on the style of their foliations. Each rock name has a specific texture that defines and distinguishes it, with their descriptions listed below.
Slate is a fine-grained metamorphic rock that exhibits a foliation called slaty cleavage that is the flat orientation of the small platy crystals of mica and chlorite forming perpendicular to the direction of stress. The minerals in slate are too small to see with the unaided eye. The thin layers in slate may resemble sedimentary bedding, but they are a result of directed stress and may lie at angles to the original strata. In fact, original sedimentary layering may be partially or completely obscured by the foliation. Thin slabs of slate are often used as a building material for roofs and tiles.
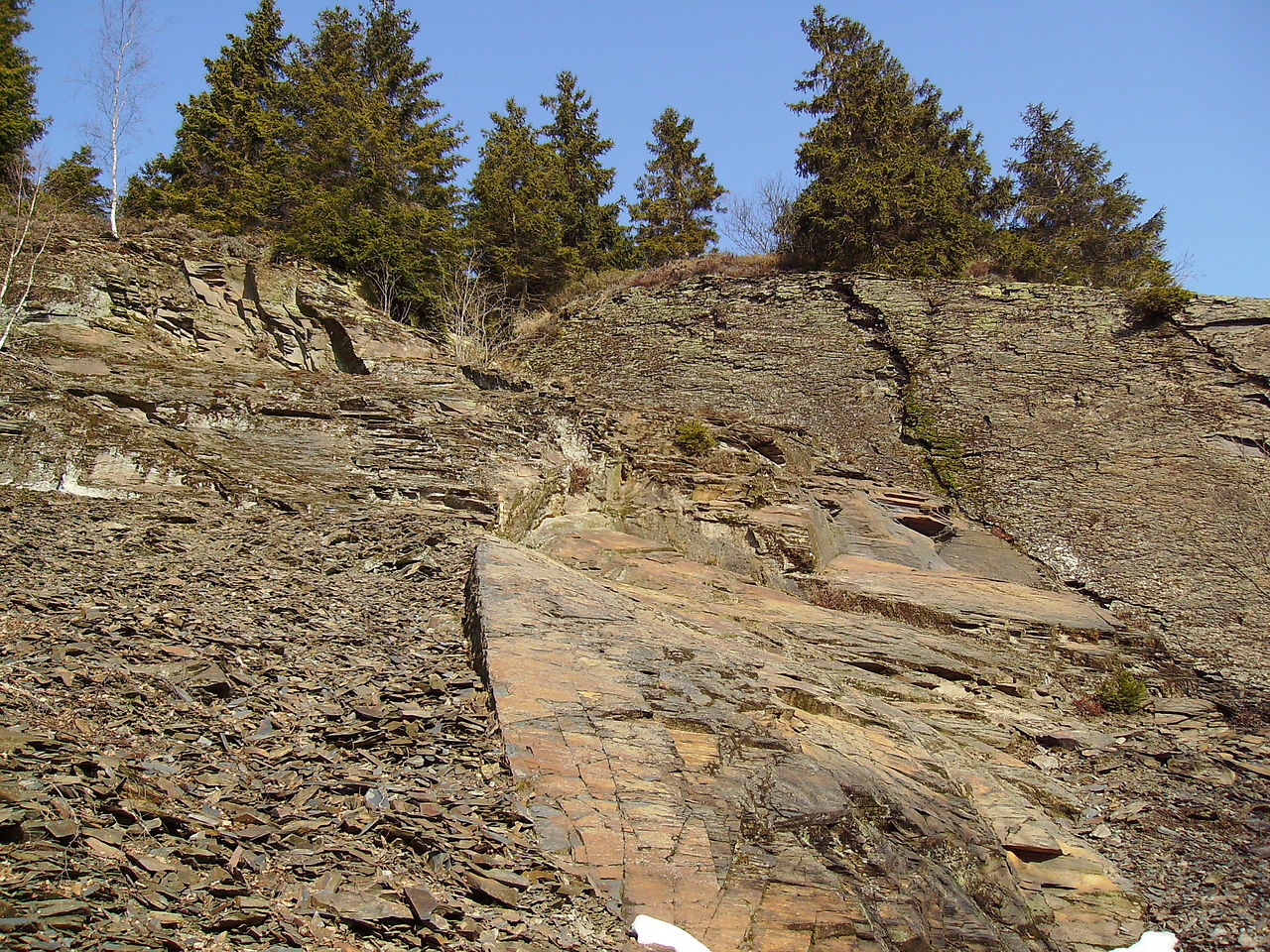
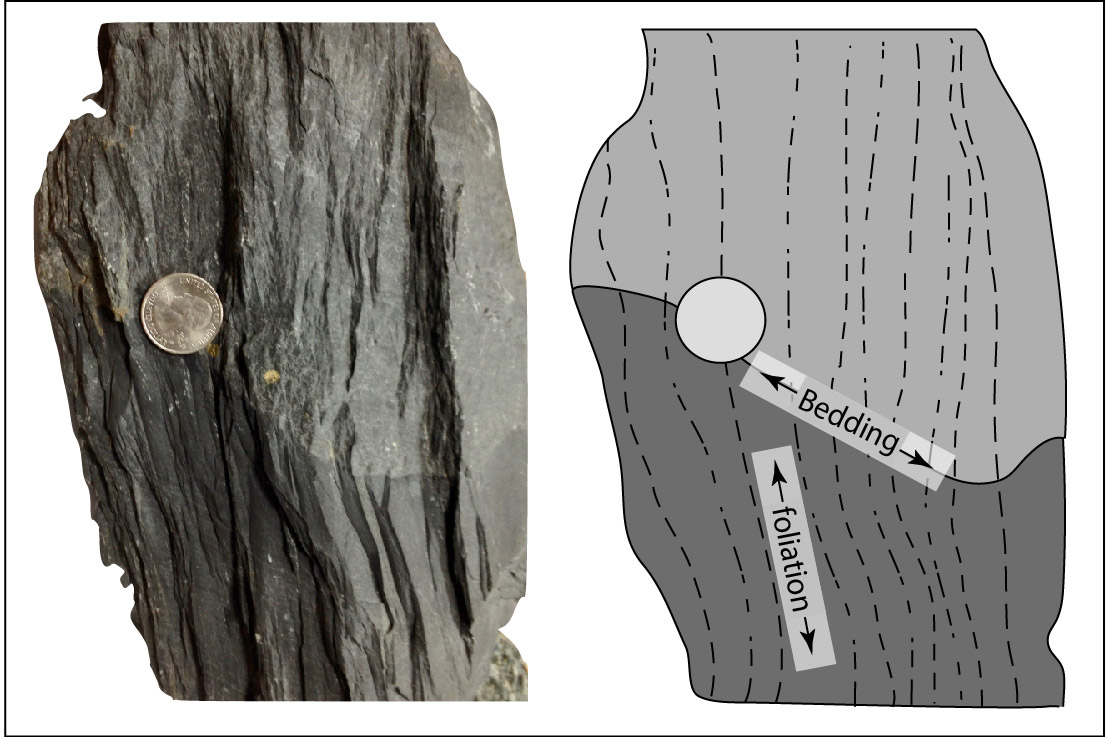
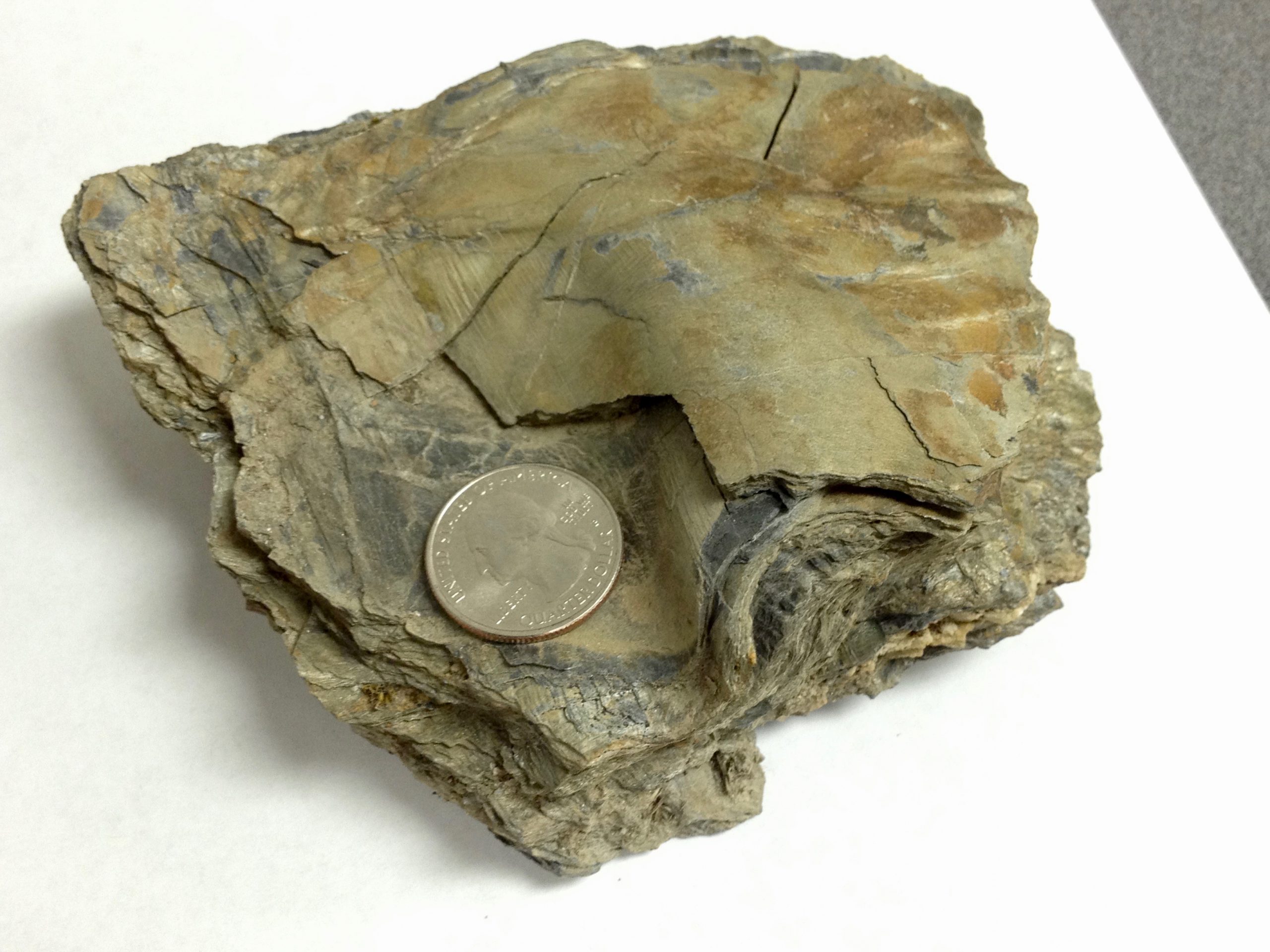
Phyllite is a foliated metamorphic rock in which platy minerals have grown larger and the surface of the foliation shows a sheen from light reflecting from the grains, perhaps even a wavy appearance, called crenulations. Similar to phyllite but with even larger grains is the foliated metamorphic rock schist, which has large platy grains visible as individual crystals. Common minerals are muscovite, biotite, and porphyroblasts of garnets. A porphyroblast is a large crystal of a particular mineral surrounded by small grains. Schistosity is a textural description of foliation created by the parallel alignment of platy visible grains. Some schists are named for their minerals such as mica schist (mostly micas), garnet schist (mica schist with garnets), and staurolite schist (mica schists with staurolite).
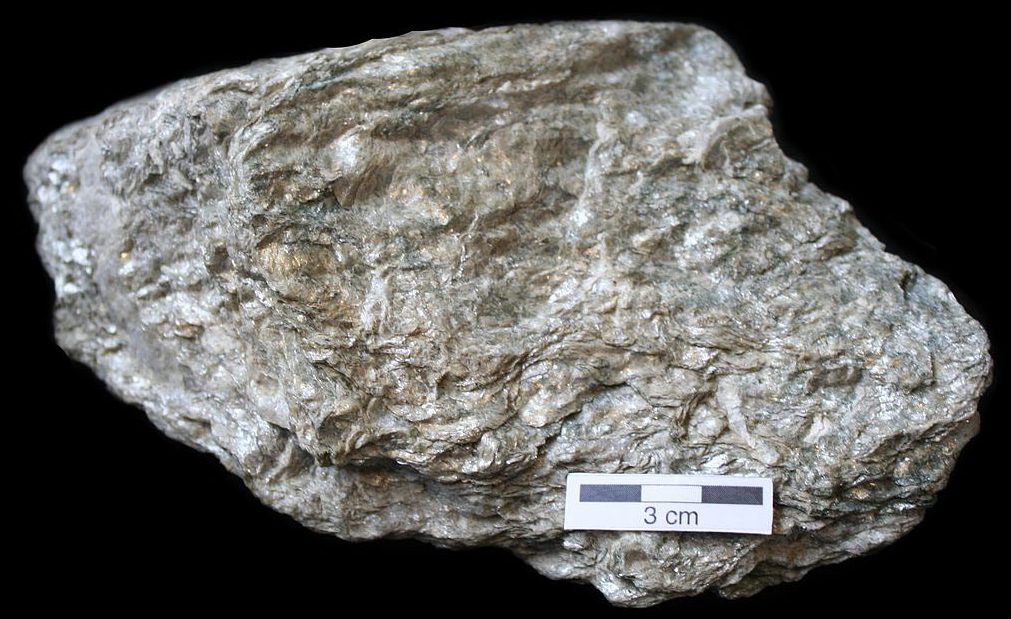
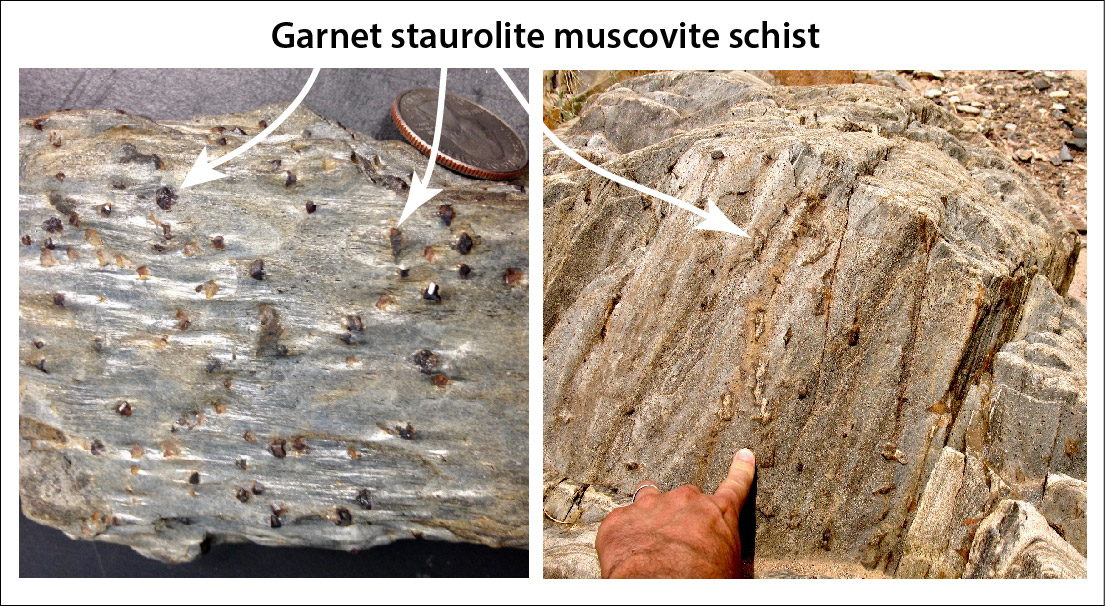
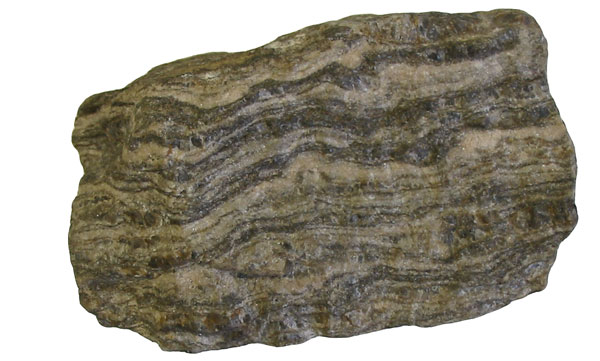
Gneissic banding is a metamorphic foliation in which visible silicate minerals separate into dark and light bands or lineations. These grains tend to be coarse and often folded. A rock with this texture is called gneiss. Since gneisses form at the highest temperatures and pressures, some partial melting may occur. This partially melted rock is a transition between metamorphic and igneous rocks called a migmatite.
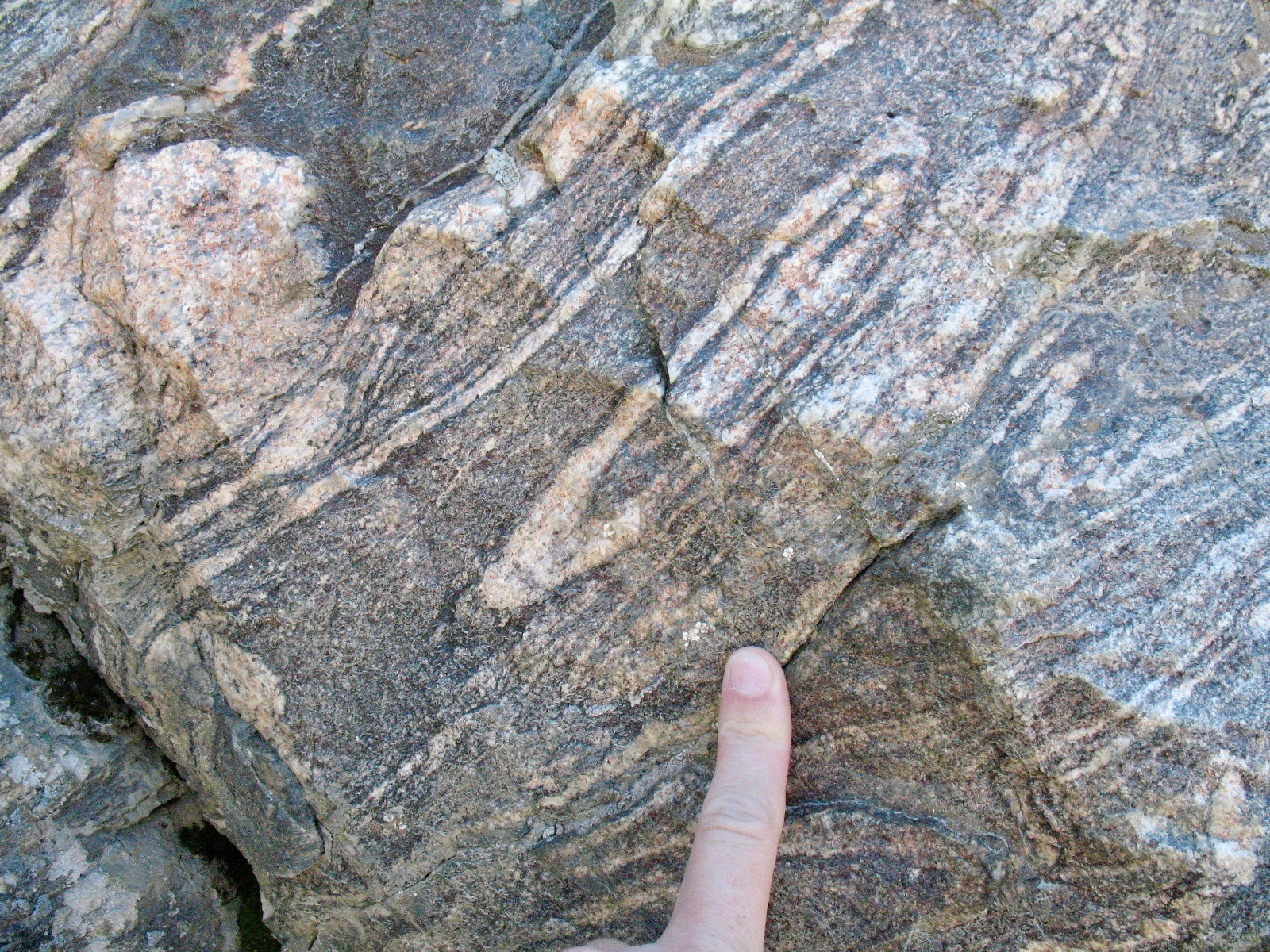
Migmatites appear as dark and light banded gneiss that may be swirled or twisted some since some minerals started to melt. Thin accumulations of light colored rock layers can occur in a darker rock that are parallel to each other, or even cut across the gneissic foliation. The lighter colored layers are interpreted to be the result of the separation of a felsic igneous melt from the adjacent highly metamorphosed darker layers, or injection of a felsic melt from some distance away.
6.2.2 Non-foliated
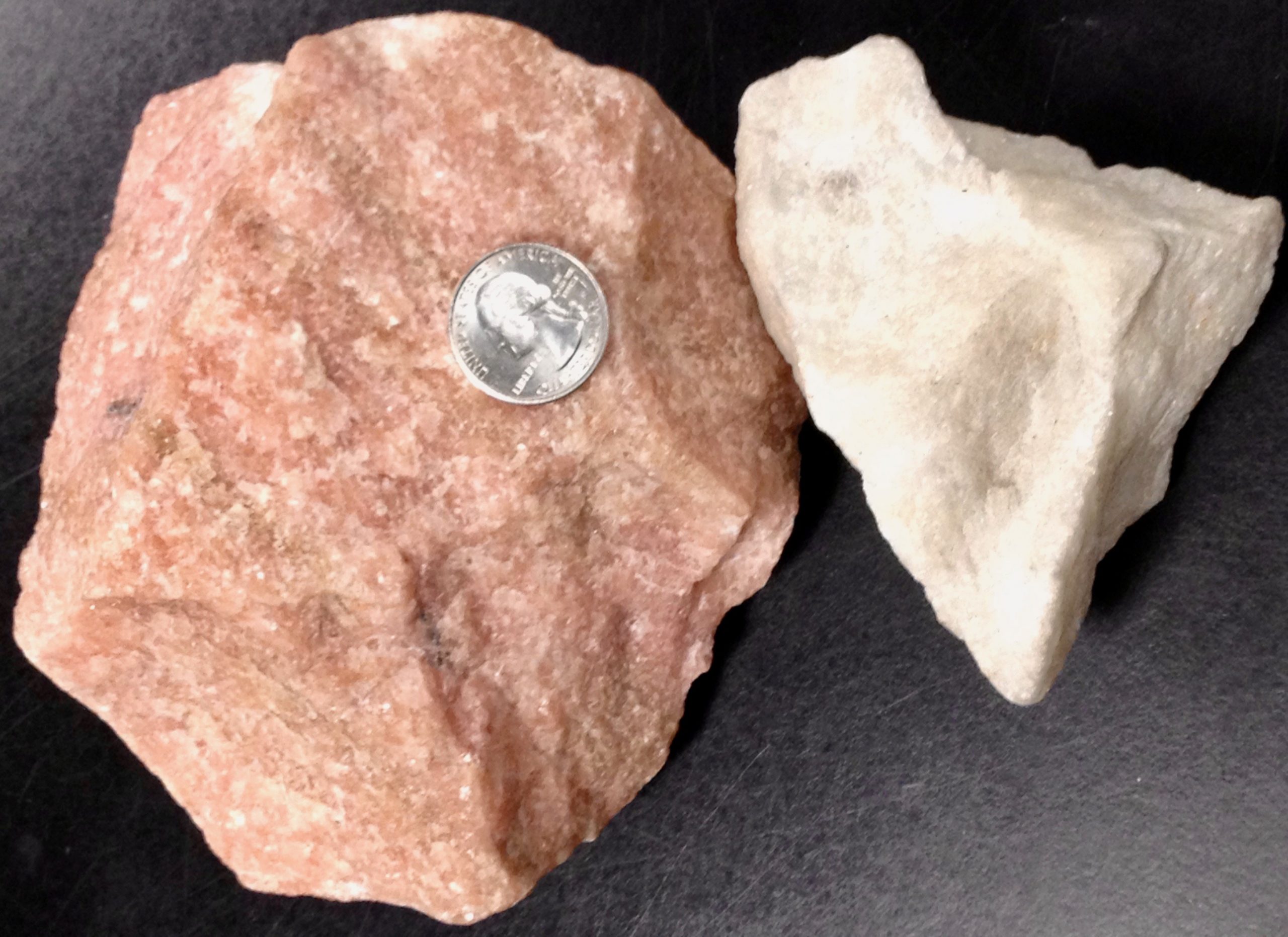
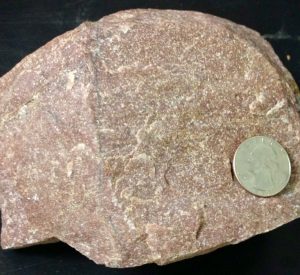
Non-foliated textures do not have lineations, foliations, or other alignments of mineral grains. Non-foliated metamorphic rocks are typically composed of just one mineral, and therefore, usually show the effects of metamorphism with recrystallization in which crystals grow together, but with no preferred direction. The two most common examples of non-foliated rocks are quartzite and marble. Quartzite is a metamorphic rock from the protolith sandstone. In quartzites, the quartz grains from the original sandstone are enlarged and interlocked by recrystallization. A defining characteristic for distinguishing quartzite from sandstone is that when broken with a rock hammer, the quartz crystals break across the grains. In a sandstone, only a thin mineral cement holds the grains together, meaning that a broken piece of sandstone will leave the grains intact. Because most sandstones are rich in quartz, and quartz is a mechanically and chemically durable substance, quartzite is very hard and resistant to weathering.
Marble is metamorphosed limestone (or dolostone) composed of calcite (or dolomite). Recrystallization typically generates larger interlocking crystals of calcite or dolomite. Marble and quartzite often look similar, but these minerals are considerably softer than quartz. Another way to distinguish marble from quartzite is with a drop of dilute hydrochloric acid. Marble will effervesce (fizz) if it is made of calcite.
A third non-foliated rock is hornfels identified by its dense, fine grained, hard, blocky or splintery texture composed of several silicate minerals. Crystals in hornfels grow smaller with metamorphism, and become so small that specialized study is required to identify them. These are common around intrusive igneous bodies and are hard to identify. The protolith of hornfels can be even harder to distinguish, which can be anything from mudstone to basalt.
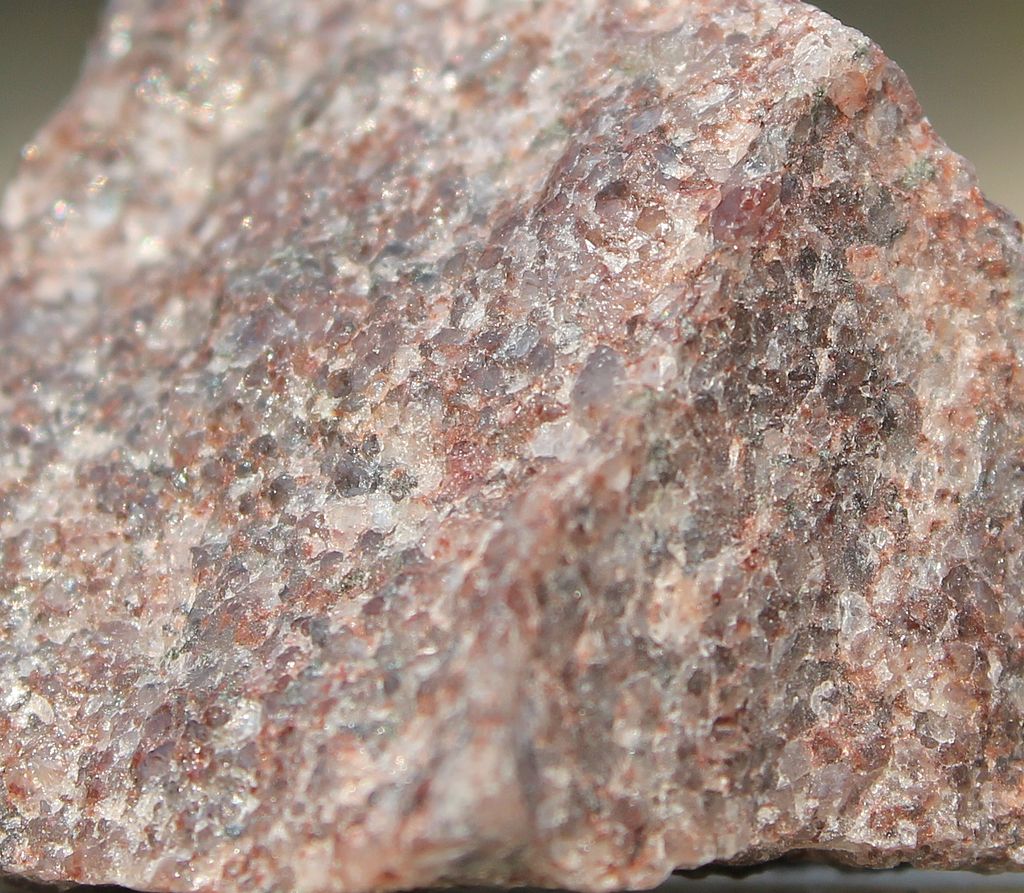
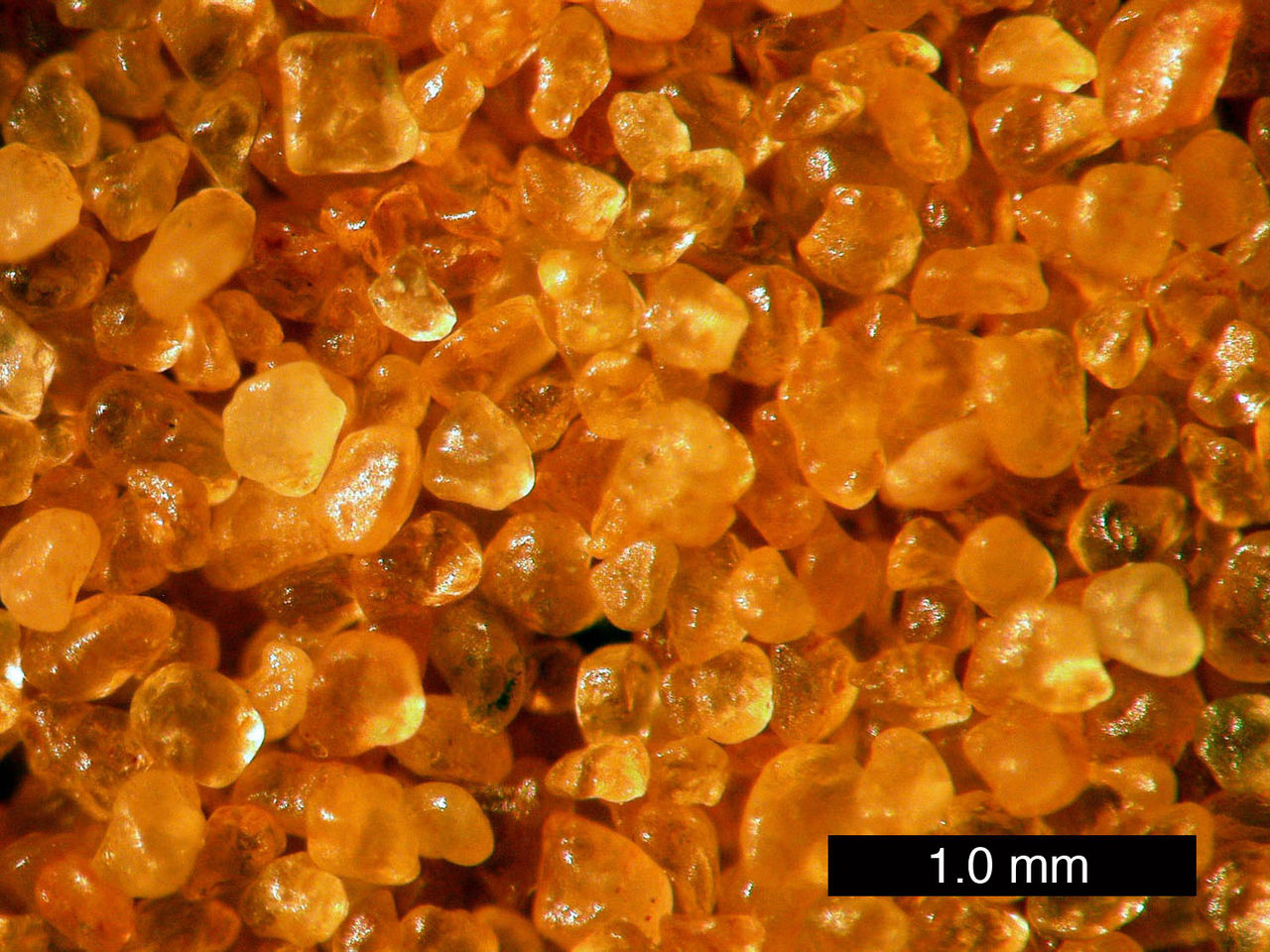
Take this quiz to check your comprehension of this section.
If you are using an offline version of this text, access the quiz for section 6.2 via the QR code.
6.3 Metamorphic Grade
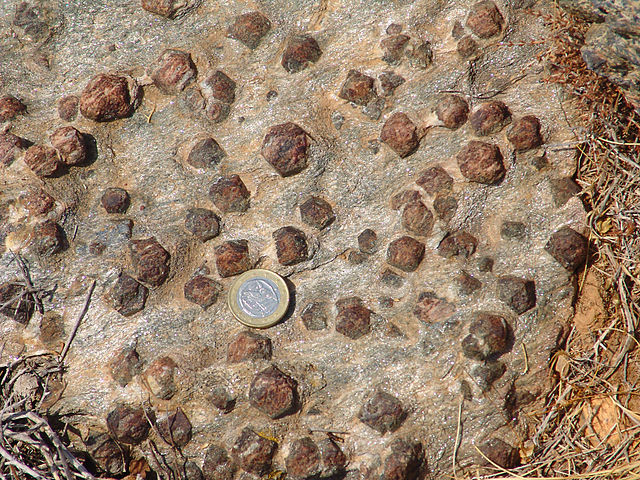
Metamorphic grade refers to the range of metamorphic change a rock undergoes, progressing from low (little metamorphic change) grade to high (significant metamorphic change) grade. Low-grade metamorphism begins at temperatures and pressures just above sedimentary rock conditions. The sequence slate→phyllite→schist→gneiss illustrates an increasing metamorphic grade.
Geologists use index minerals that form at certain temperatures and pressures to identify metamorphic grade. These index minerals also provide important clues to a rock’s sedimentary protolith and the metamorphic conditions that created it. Chlorite, muscovite, biotite, garnet, and staurolite are index minerals representing a respective sequence of low-to-high grade rock. The figure shows a phase diagram of three index minerals—sillimanite, kyanite, and andalusite—with the same chemical formula (Al2SiO5) but having different crystal structures (polymorphism) created by different pressure and temperature conditions.
Complete this interactive activity to check your understanding.
If you are using an offline version of this text, access this interactive activity via the QR code.
Some metamorphic rocks are named based on the highest grade of index mineral present. Chlorite schist includes the low-grade index mineral chlorite. Muscovite schist contains the slightly higher grade muscovite, indicating a greater degree of metamorphism. Garnet schist includes the high grade index mineral garnet, and indicating it has experienced much higher pressures and temperatures than chlorite.
Take this quiz to check your comprehension of this section.
If you are using an offline version of this text, access the quiz for section 6.3 via the QR code.
6.4 Metamorphic Environments
As with igneous processes, metamorphic rocks form at different zones of pressure (depth) and temperature as shown on the pressure-temperature (P-T) diagram. The term facies is an objective description of a rock. In metamorphic rocks facies are groups of minerals called mineral assemblages. The names of metamorphic facies on the pressure-temperature diagram reflect minerals and mineral assemblages that are stable at these pressures and temperatures and provide information about the metamorphic processes that have affected the rocks. This is useful when interpreting the history of a metamorphic rock.
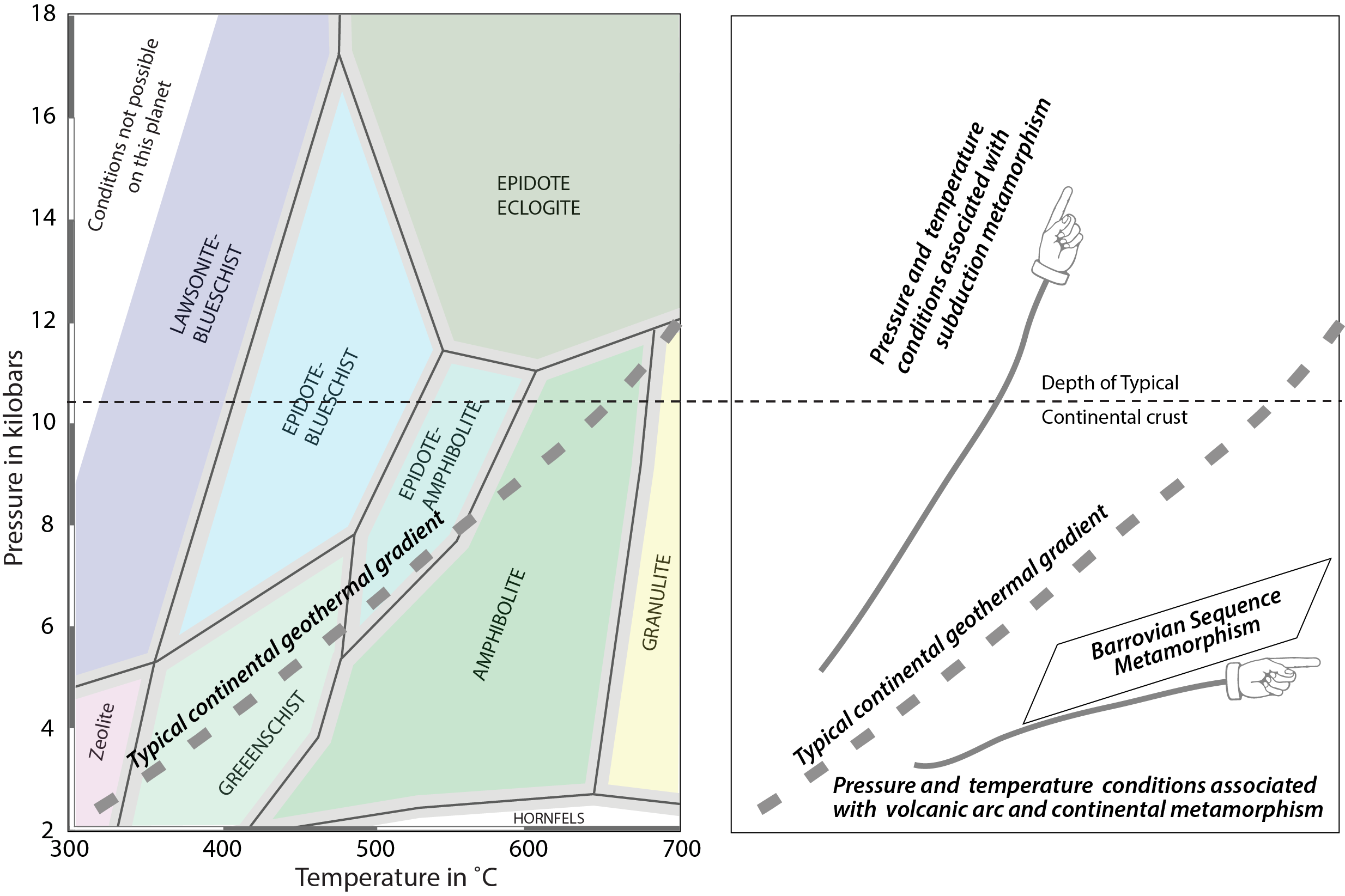
In the late 1800s, British geologist George Barrow mapped zones of index minerals in different metamorphic zones of an area that underwent regional metamorphism. Barrow outlined a progression of index minerals, named the Barrovian Sequence, that represents increasing metamorphic grade: chlorite (slates and phyllites) -> biotite (phyllites and schists) -> garnet (schists) -> staurolite (schists) -> kyanite (schists) -> sillimanite (schists and gneisses).
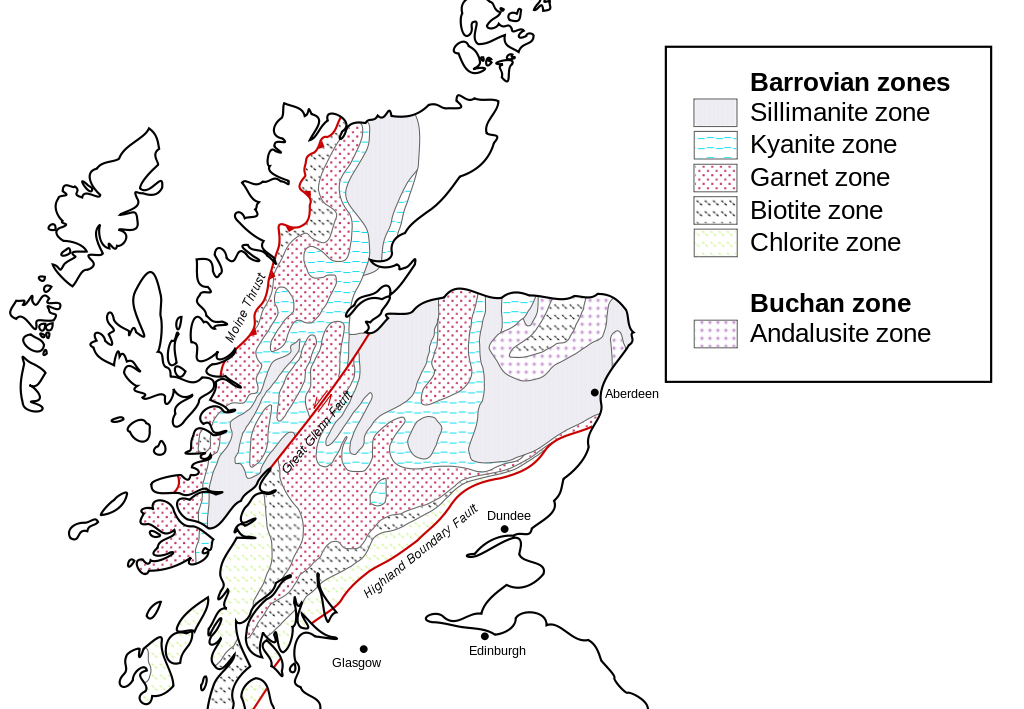
The first of the Barrovian sequence has a mineral group that is commonly found in the metamorphic greenschist facies. Greenschist rocks form under relatively low pressure and temperatures and represent the fringes of regional metamorphism. The “green” part of the name is derived from green minerals like chlorite, serpentine, and epidote, and the “schist” part is applied due to the presence of platy minerals such as muscovite.
Many different styles of metamorphic facies are recognized, tied to different geologic and tectonic processes. Recognizing these facies is the most direct way to interpret the metamorphic history of a rock. A simplified list of major metamorphic facies is given below.
6.4.1 Burial Metamorphism
Burial metamorphism occurs when rocks are deeply buried, at depths of more than 2000 meters (1.24 miles). Burial metamorphism commonly occurs in sedimentary basins, where rocks are buried deeply by overlying sediments. As an extension of diagenesis, a process that occurs during lithification (chapter 5), burial metamorphism can cause clay minerals, such as smectite, in shales to change to another clay mineral illite. Or it can cause quartz sandstone to metamorphose into the quartzite such the Big Cottonwood Formation in the Wasatch Range of Utah. This formation was deposited as ancient near-shore sands in the late Proterozoic (see chapter 7), deeply buried and metamorphosed to quartzite, folded, and later exposed at the surface in the Wasatch Range today. Increase of temperature with depth in combination with an increase of confining pressure produces low-grade metamorphic rocks with a mineral assemblages indicative of a zeolite facies.
6.4.2 Contact Metamorphism
Contact metamorphism occurs in rock exposed to high temperature and low pressure, as might happen when hot magma intrudes into or lava flows over pre-existing protolith. This combination of high temperature and low pressure produces numerous metamorphic facies. The lowest pressure conditions produce hornfels facies, while higher pressure creates greenschist, amphibolite, or granulite facies.
As with all metamorphic rock, the parent rock texture and chemistry are major factors in determining the final outcome of the metamorphic process, including what index minerals are present. Fine-grained shale and basalt, which happen to be chemically similar, characteristically recrystallize to produce hornfels. Sandstone (silica) surrounding an igneous intrusion becomes quartzite via contact metamorphism, and limestone (carbonate) becomes marble.
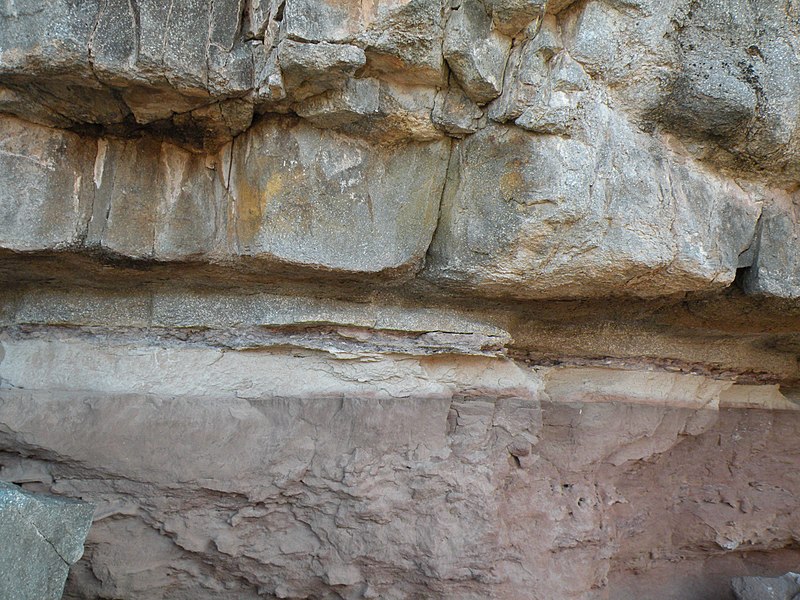
When contact metamorphism occurs deeper in the Earth, metamorphism can be seen as rings of facies around the intrusion, resulting in aureoles. These differences in metamorphism appear as distinct bands surrounding the intrusion, as can be seen around the Alta Stock in Little Cottonwood Canyon, Utah. The Alta Stock is a granite intrusion surrounded first by rings of the index minerals amphibole (tremolite) and olivine (forsterite), with a ring of talc (dolostone) located further away.
6.4.3 Regional Metamorphism
Regional metamorphism occurs when parent rock is subjected to increased temperature and pressure over a large area, and is often located in mountain ranges created by converging continental crustal plates. This is the setting for the Barrovian sequence of rock facies, with the lowest grade of metamorphism occurring on the flanks of the mountains and highest grade near the core of the mountain range, closest to the convergent boundary.
An example of an old regional metamorphic environment is visible in the northern Appalachian Mountains while driving east from New York state through Vermont and into New Hampshire. Along this route the degree of metamorphism gradually increases from sedimentary parent rock, to low-grade metamorphic rock, then higher-grade metamorphic rock, and eventually the igneous core. The rock sequence is sedimentary rock, slate, phyllite, schist, gneiss, migmatite, and granite. In fact, New Hampshire is nicknamed the Granite State. The reverse sequence can be seen heading east, from eastern New Hampshire to the coast.
6.4.4 Subduction Zone Metamorphism
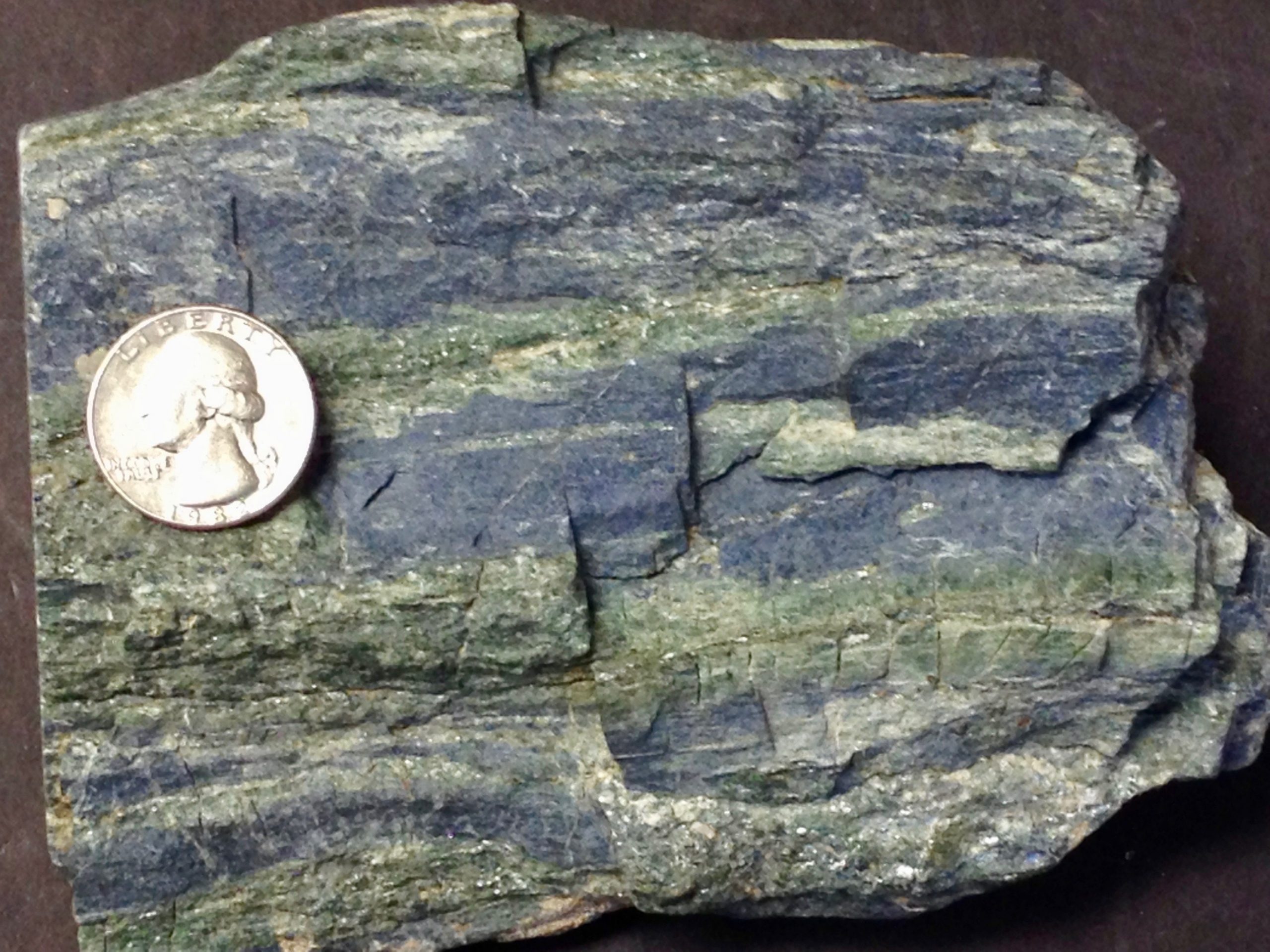
Subduction zone metamorphism is a type of regional metamorphism that occurs when a slab of oceanic crust is subducted under continental crust (see chapter 2). Because rock is a good insulator, the temperature of the descending oceanic slab increases slowly relative to the more rapidly increasing pressure, creating a metamorphic environment of high pressure and low temperature. Glaucophane, which has a distinctive blue color, is an index mineral found in blueschist facies (see metamorphic facies diagram). The California Coast Range near San Francisco has blueschist–facies rocks created by subduction-zone metamorphism, which include rocks made of blueschist, greenstone, and red chert. Greenstone, which is metamorphized basalt, gets its color from the index mineral chlorite.
6.4.5 Fault Metamorphism
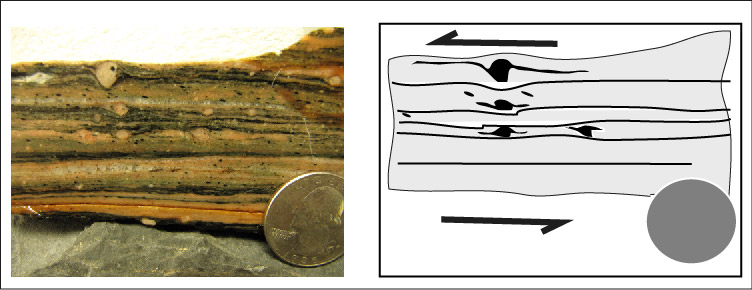
There are a range of metamorphic rocks made along faults. Near the surface, rocks are involved in repeated brittle faulting produce a material called rock flour, which is rock ground up to the particle size of flour used for food. At lower depths, faulting create cataclastites, chaotically-crushed mixes of rock material with little internal texture. At depths below cataclasites, where strain becomes ductile, mylonites are formed. Mylonites are metamorphic rocks created by dynamic recrystallization through directed shear forces, generally resulting in a reduction of grain size. When larger, stronger crystals (like feldspar, quartz, garnet) embedded in a metamorphic matrix are sheared into an asymmetrical eye-shaped crystal, an augen is formed.
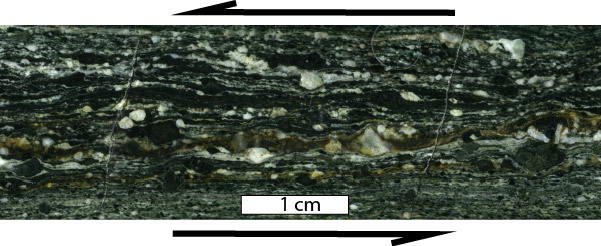
6.4.6 Shock Metamorphism
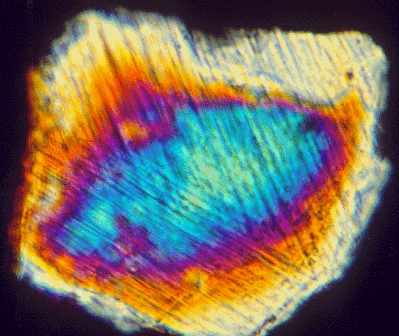
Shock (also known as impact) metamorphism is metamorphism resulting from meteor or other bolide impacts, or from a similar high-pressure shock event. Shock metamorphism is the result of very high pressures (and higher, but less extreme temperatures) delivered relatively rapidly. Shock metamorphism produces planar deformation features, tektites, shatter cones, and quartz polymorphs. Shock metamorphism produces planar deformation features (shock laminae), which are narrow planes of glassy material with distinct orientations found in silicate mineral grains. Shocked quartz has planar deformation features.
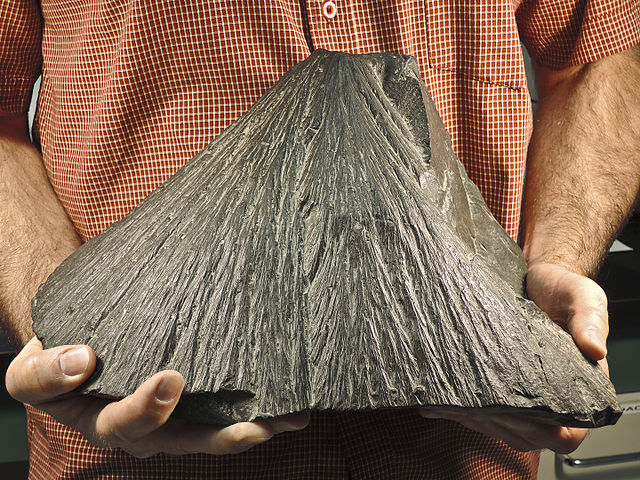
Shatter cones are cone-shaped pieces of rock created by dynamic branching fractures caused by impacts. While not strictly a metamorphic structure, they are common around shock metamorphism. Their diameter can range from microscopic to several meters. Fine-grained rocks with shatter cones show a distinctive horsetail pattern.
Shock metamorphism can also produce index minerals, though they are typically only found via microscopic analysis. The quartz polymorphs coesite and stishovite are indicative of impact metamorphism. As discussed in chapter 3, polymorphs are minerals with the same composition but different crystal structures. Intense pressure (> 10 GPa) and moderate to high temperatures (700-1200 °C) are required to form these minerals.
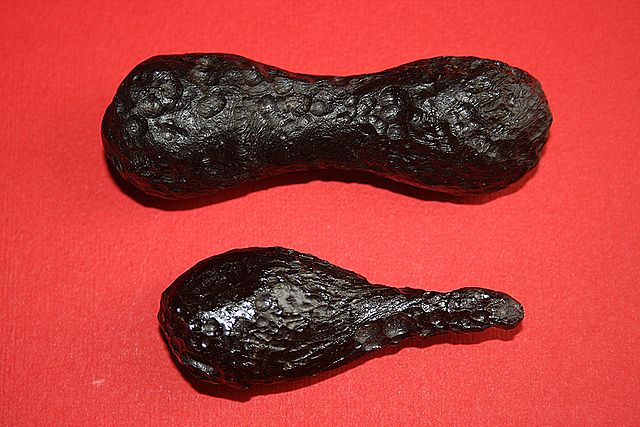
Shock metamorphism can also produce glass. Tektites are gravel-size glass grains ejected during an impact event. They resemble volcanic glass but, unlike volcanic glass, tektites contain no water or phenocrysts, and have a different bulk and isotopic chemistry. Tektites contain partially melted inclusions of shocked mineral grains. Although all are melt glasses, tektites are also chemically distinct from trinitite, which is produced from thermonuclear detonations, and fulgurites, which are produced by lightning strikes. All geologic glasses not derived from volcanoes can be called with the general term pseudotachylytes, a name which can also be applied to glasses created by faulting. The term pseudo in this context means ‘false’ or ‘in the appearance of’, a volcanic rock called tachylite because the material observed looks like a volcanic rock, but is produced by significant shear heating.
Take this quiz to check your comprehension of this section.
If you are using an offline version of this text, access the quiz for section 6.4 via the QR code.
Video 6.1: Identifying metamorphic rocks.
If you are using an offline version of this text, access this YouTube video via the QR code.
Video 6.2: Identifying metamorphic rocks.
If you are using an offline version of this text, access this YouTube video via the QR code.
Summary
Metamorphism is the process that changes existing rocks (called protoliths) into new rocks with new minerals and new textures. Increases in temperature and pressure are the main causes of metamorphism, with fluids adding important mobilization of materials. The primary way metamorphic rocks are identified is with texture. Foliated textures come from platy minerals forming planes in a rock, while non-foliated metamorphic rocks have no internal fabric. Grade describes the amount of metamorphism in a rock, and facies are a set of minerals that can help guide an observer to an interpretation of the metamorphic history of a rock. Different tectonic or geologic environments cause metamorphism, including collisions, subduction, faulting, and even impacts from space.
Take this quiz to check your comprehension of this chapter.
If you are using an offline version of this text, access the quiz for chapter 6 via the QR code.
Text References
- Bucher, K., and Grapes, R., 2011, Petrogenesis of metamorphic rocks: Springer, 341 p.
- Jeong, I.-K., Heffner, R.H., Graf, M.J., and Billinge, S.J.L., 2003, Lattice dynamics and correlated atomic motion from the atomic pair distribution function: Phys. Rev. B Condens. Matter, v. 67, no. 10, p. 104301.
- Marshak, S., 2009, Essentials of Geology, 3rd or 4th Edition.
- Proctor, B.P., McAleer, R., Kunk, M.J., and Wintsch, R.P., 2013, Post-Taconic tilting and Acadian structural overprint of the classic Barrovian metamorphic gradient in Dutchess County, New York: Am. J. Sci., v. 313, no. 7, p. 649–682.
- Timeline of Art History, 2007, Reference Reviews, v. 21, no. 8, p. 45–45.
Figure References
Figure 6.1: Rock cycle showing the five materials (such as igneous rocks and sediment) and the processes by which one changes into another (such as weathering). Kindred Grey. 2022. CC BY 4.0.
Figure 6.2: Difference between pressure and stress and how they deform rocks. Peter Davis. 2017. CC BY-NC-SA 4.0. https://slcc.pressbooks.pub/introgeology/chapter/6-metamorphic-rocks/
Figure 6.3: Pebbles (that used to be spherical or close to spherical) in quartzite deformed by directed stress. Peter Davis. 2017. CC BY-NC-SA 4.0. https://slcc.pressbooks.pub/introgeology/chapter/6-metamorphic-rocks/
Figure 6.4: An igneous rock granite (left) and foliated high-temperature and high-pressure metamorphic rock gneiss (right) illustrating a metamorphic texture. Peter Davis. 2017. CC BY-NC-SA 4.0. https://slcc.pressbooks.pub/introgeology/chapter/6-metamorphic-rocks/
Figure 6.5: Black smoker hydrothermal vent with a colony of giant (6’+) tube worms. NOAA. 2006. Public domain. https://commons.wikimedia.org/wiki/File:Main_Endeavour_black_smoker.jpg
Table 6.1: Metamorphic rock identification table. Kindred Grey. 2022. Table data from Belinda Madsen’s graphic in CH 6 of An Introduction to Geology. OpenStax. Salt Lake Community College. CC BY-NC-SA 4.0.
Figure 6.6: Example of lineation where minerals are aligned like a stack of straws or pencils. Peter Davis. 2017. CC BY-NC-SA 4.0. https://slcc.pressbooks.pub/introgeology/chapter/6-metamorphic-rocks/
Figure 6.7: An example of foliation WITH lineation. Peter Davis. 2017. CC BY-NC-SA 4.0. https://slcc.pressbooks.pub/introgeology/chapter/6-metamorphic-rocks/
Figure 6.8: An example of foliation WITHOUT lineation. Peter Davis. 2017. CC BY-NC-SA 4.0. https://slcc.pressbooks.pub/introgeology/chapter/6-metamorphic-rocks/
Figure 6.9: Rock breaking along flat even planes. Uta Baumfelder. 2010. Public domain. https://commons.wikimedia.org/wiki/File:Ehemaliger_Schiefertagebau_am_Brand.JPG
Figure 6.10: Foliation versus bedding. Peter Davis. 2017. CC BY-NC-SA 4.0. https://slcc.pressbooks.pub/introgeology/chapter/6-metamorphic-rocks/
Figure 6.11: Phyllite with a small fold. Peter Davis. 2017. CC BY-NC-SA 4.0. https://slcc.pressbooks.pub/introgeology/chapter/6-metamorphic-rocks/
Figure 6.12: Schist. Michael C. Rygel. 2012. CC BY-SA 3.0. https://commons.wikimedia.org/wiki/File:Schist_detail.jpg
Figure 6.13: Garnet staurolite muscovite schist. Peter Davis. 2017. CC BY-NC-SA 4.0. https://slcc.pressbooks.pub/introgeology/chapter/6-metamorphic-rocks/
Figure 6.14: Gneiss. Siim Sepp. 2005. CC BY-SA 3.0. https://commons.wikimedia.org/wiki/File:Gneiss.jpg
Figure 6.15: Migmatite, a rock which was partially molten. Peter Davis. 2017. CC BY-NC-SA 4.0. https://slcc.pressbooks.pub/introgeology/chapter/6-metamorphic-rocks/
Figure 6.16: Marble. Peter Davis. 2017. CC BY-NC-SA 4.0. https://slcc.pressbooks.pub/introgeology/chapter/6-metamorphic-rocks/
Figure 6.17: Baraboo quartzite. Peter Davis. 2017. CC BY-NC-SA 4.0. https://slcc.pressbooks.pub/introgeology/chapter/6-metamorphic-rocks/
Figure 6.18: Macro view of quartzite. Manishwiki15. 2012. CC BY-SA 3.0. https://commons.wikimedia.org/wiki/File:Sample_of_Quartzite.JPG
Figure 6.19: Unmetamorphosed, unconsolidated sand grains have space between the grains. Wilson44691. 2008. Public domain. https://commons.wikimedia.org/wiki/File:CoralPinkSandDunesSand.JPG
Figure 6.20: Garnet schist. Graeme Churchard (GOC53). 2005. CC BY 2.0. https://commons.wikimedia.org/wiki/File:Garnet_Mica_Schist_Syros_Greece.jpg
Figure 6.21: Pressure–temperature graphs of various metamorphic facies. Peter Davis. 2017. CC BY-NC-SA 4.0. https://slcc.pressbooks.pub/introgeology/chapter/6-metamorphic-rocks/
Figure 6.22: Barrovian sequence in Scotland. Woudloper. 2009. CC BY-SA 3.0. https://commons.wikimedia.org/wiki/File:Scotland_metamorphic_zones_EN.svg
Figure 6.23: Contact metamorphism in outcrop. Random Tree. 2012. Public domain. https://commons.wikimedia.org/wiki/File:Metamorphic_Aureole_in_the_Henry_Mountains.JPG
Figure 6.24: Blueschist. Peter Davis. 2017. CC BY-NC-SA 4.0. https://slcc.pressbooks.pub/introgeology/chapter/6-metamorphic-rocks/
Figure 6.25: Mylonite. Peter Davis. 2017. CC BY-NC-SA 4.0. https://slcc.pressbooks.pub/introgeology/chapter/6-metamorphic-rocks/
Figure 6.26: Examples of augens. Peter Davis. 2017. CC BY-NC-SA 4.0. https://slcc.pressbooks.pub/introgeology/chapter/6-metamorphic-rocks/
Figure 6.27: Shock lamellae in a quartz grain. Glen A. Izett. 2000. Public domain. https://commons.wikimedia.org/wiki/File:820qtz.jpg
Figure 6.28: Shatter cone. JMGastonguay. 2014. CC BY-SA 4.0. https://commons.wikimedia.org/wiki/File:ShatterConeCharlevoix1.jpg
Figure 6.29: Tektites. Brocken Inaglory. 2007. CC BY-SA 3.0. https://en.wikipedia.org/wiki/File:Two_tektites.JPG
The measure of the vibrational (kinetic) energy of a substance.
Rocks and minerals that change within the Earth are called metamorphic, changed by heat and pressure. Metamorphism is the name of the process.
The process of changing a mineral without melting.
A natural substance that is typically solid, has a crystalline structure, and is typically formed by inorganic processes. Minerals are the building blocks of most rocks.
A planar alignment of minerals and textures within a rock.
Metamorphic rock with a strong foliation but no visible minerals, derived from mudstones or shales.
A rock more metamorphosed than slate, to the point that microscopic (but larger) mica gives the rock a glow, called a sheen. Crenulation, or small bends/folds in the foliation can be present.
Rock more metamorphosed than phyllite, to the point that mica grains are visible. Larger porphyroblasts are sometimes present.
A very high grade metamorphic rock, higher grade than schist, with a separation of light and dark minerals.
Minerals that form at a specific range of temperatures and pressures. Using a collection of index minerals narrows down the conditions of rock formation.
A specific set of index minerals tied to specific styles of metamorphism. When these minerals are present, it allows a history of metamorphism to be determined.
A solid part of the lithosphere which moves as a unit, i.e. the entire plate generally moves the same direction at the same speed.
The theory that the outer layer of the Earth (the lithosphere) is broken in several plates, and these plates move relative to one another, causing the major topographic features of Earth (e.g. mountains, oceans) and most earthquakes and volcanoes.
A zone of contact metamorphism that surrounds an intrusion. Since intrusions are typically somewhat round in cross section, the pattern of metamorphism is concentric about the intrusion.
Metamorphism that occurs when rocks are next to a hot intrusion of magma.
Metamorphism which occurs with hot fluids going within rocks, altering and changing the rocks.
Valuable material in the Earth, typically used for metallic mineral resources.
The concept that any rock type (igneous, sedimentary, and metamorphic) can change into another rock type under the right conditions over geologic time.
Rocks formed via heat and/or pressure which change the minerals within the rock.
Rocks that are formed from liquid rock, i.e. from volcanic processes.
The mineral makeup of a rock, i.e. which minerals are found within a rock.
Arrangement of minerals within a rock.
A rock that contains material which can be turned into petroleum resources. Organic-rich muds form good source rocks.
The rocks that existed before the changes that lead to a metamorphic rock, i.e. what rock would exist if the metamorphism was reversed.
The outermost chemical layer of the Earth, defined by its low density and higher concentrations of lighter elements. The crust has two types: continental, which is the thick, more ductile, and lowest density, and oceanic, which is higher density, more brittle, and thinner.
Non-directional pressure resulting from burial.
Stress that has a strong directional component (unequal), typically creating elongated or flattened features.
A positively-charged ion. In geology, this commonly includes ions of the elements Ca+2, Na+1, K+1, Fe+2,+3, Al+3, and Mg+2.
Rocks that are formed by sedimentary processes, including sediments lithifying and precipitation from solution.
Liquid rock within the Earth.
The average change in temperature that is experienced as material moves into the Earth. Near the surface, this rate is about 25°C/km.
Force applied to an object, typically dealing with forces within the Earth.
The deformation that results from application of a stress.
Large, easy-to-see crystals within an igneous rock. This is common in intrusive rocks.
General name of a felsic rock that is intrusive. Has more felsic minerals than mafic minerals.
The average diameter of a grain of sediment, ranging from small, also known as fine-grained (e.g. clay, silt) to large, also known as coarse-grained (e.g. boulder).
The process in which solids (like minerals) are disassociated and the ionic components are dispersed in a liquid (usually water).
The act of a solid coming out of solution, typically resulting from a drop in temperature or a decrease of the dissolving material.
Planar feature where two blocks of bedrock move past each other via earthquakes.
A group of all atoms with a specific number of protons, having specific, universal, and unique properties.
Place where material is extracted from the Earth for human use.
A down-warped feature in the crust.
Minerals bonded via a sulfur (S-2) atom.
Minerals made from just a single element, bonded to itself. Examples include gold, silver, copper, and diamond, which is a native version of carbon.
Term for the underlying lithified rocks that make up the geologic record in an area. This term can sometimes refer to only the deeper, crystalline (non-layered) rocks.
A chemical or biochemical rock made of mainly calcite.
Can refer to a volcanic rock with lower silica composition, or the minerals that make up those rocks, namely olivine, pyroxene, amphibole, and biotite. Mafic rocks are darker in color and contain more minerals that are dark in color, but can contain some plagioclase feldspar. Primary mafic rocks are basalt (extrusive) and gabbro (intrusive).
Middle chemical layer of the Earth, made of mainly iron and magnesium silicates. It is generally denser than the crust (except for older oceanic crust) and less dense than the core.
(Fe,Mg)2SiO4. Typically translucent olive green and equant, with no cleavage. Common in mafic igneous rocks and in the mantle, but easily weathered in surface conditions. Structure is isolated silica tetrahedra. Known as peridot when a gem.
General name of a mafic rock that is extrusive. Generally has a black groundmass color.
Rock formed from hydrothermal alteration of basalt, made of serpentine.
A divergent boundary within an oceanic plate, where new lithosphere and crust is created as the two plates spread apart. Mid-ocean ridge and spreading center are synonyms.
The thin, outer layer of the Earth which makes up the rocky bottom of the ocean basins. Oceanic crust is much thinner (but denser) than continental crust. Oceanic crust is made of rocks similar to basalt and as it cools, becomes more dense.
A break within a rock that has no relative movement between the sides. Caused by cooling, pressure release, tectonic forces, etc.
Mineral group in which the silica tetrahedra, SiO4-4, is the building block.
Mineral chimneys that form at hydrothermal vents.
Opening of a volcano where lava can erupt.
Metamorphic textures that do not have a directional component of its minerals.
X1A2-3Z4O10(OH, F)2, where commonly X=K, Na, Ca; A=Al, Mg, Fe; Z=Si, Al. Has two more-common occurrences, light-colored (translucent and pearly tan) muscovite, and dark-colored biotite. Has one strong cleavage, and is typically seen as sheets, in stacks or "books." Common in many igneous and metamorphic rocks. Structure is two-dimensional sheets of silica tetrahedra in a hexagonal network.
Linear alignment of minerals within a rock.
SiO2. Transparent, but can be any color imaginable with impurities. No cleavage, hard, and commonly forms equant masses. Perfect crystals are hexagonal prisms topped with pyramidal shapes. One of the most common minerals, and is found in many different geologic settings, including the dominant component of sand on the surface of Earth. Structure is a three-dimensional network of silica tetrahedra, connected as much as possible to each other.
A microscopic foliation in slate, in which flat slabs and planes of rock develop.
Discernible layers of rock, typically from a sedimentary rock.
Term for coarse grained, visible, platy minerals in a planar fabric, typical of schists.
A separation of light (felsic) and dark (mafic) minerals in higher grade metamorphic rocks like gneiss.
The process of some material being derived from a heterogenous mixture when melting (e.g. rocks). Because all rocks are made of many different components, they have many different melting points. As they are heated, certain easy-to-melt components will be melted first.
A rock transitional between metamorphic and igneous rock, i.e. rocks so metamorphosed that they begin the process of melting.
Can refer to a volcanic rock with higher silica composition, or the minerals that make up those rocks, namely quartz, feldspar, and muscovite mica. Felsic rocks are lighter in color and contain more minerals that are light in color. Primary felsic rocks are rhyolite (extrusive) and granite (intrusive).
A metamorphosed sandstone.
A metamorphosed limestone.
A rock primarily made of sand.
Breaking down rocks into small pieces by chemical or mechanical means.
CaCO3. Pure form is clear, but can take on many different colors with impurities. It is soft, fizzes in acid, and has three cleavages that are not at 90°.
A dense, hard metamorphic rock, typically derived from contact metamorphism.
Igneous rock cooling, and thus forming, inside of the Earth, i.e. under the surface.
A rock made of primarily mud, i.e. particles smaller than sand (≤0.064 mm).
A qualitative measure of the amount of metamorphism that has occurred or the amount of a resource present in an ore.
Chart that show the stability of different phases of a substance at different conditions.
A specific chemical composition that forms different minerals and different temperatures and pressures. Quartz has several different polymorphs, including coesite, tridimite, and stishovite.
A specific set of features that are tied together in an interpretive group. Facies can be based on mineralogy, biologic factors, fossils, rock types, etc.
An observation that is completely free of bias, i.e. anyone and everyone would make the same observation.
Metamorphism that occurs with large-scale tectonic processes, like collision zones.
Metamorphism that is caused by confining pressure and heat, both increasing with depth.
A local or regional depression which allows sediments to accumulate.
Pieces of rock that have been weathered and possibly eroded.
Stresses that pull objects apart into a larger surface area or volume; stretching forces.
Changes in sedimentary rocks due to increased (but low when compared to metamorphism) temperatures and pressures. This can include deposition of new minerals (e.g. limestone converting to dolomite) or dissolution of existing minerals.
The process of turning sediment into sedimentary rock, including deposition, compaction, and cementation.
A very fine-grained rock with very thin layering (fissile).
An extensive, distinct, and mapped set of geologic layers.
The part of the coastline which is directly related to water-land interaction, specifically the tidal zone and the range of wave base.
Meaning "earlier life," the third eon of Earth's history, starting at 2.5 billion years ago and ending at 541 million years ago. Marked by increasing atmospheric oxygen and the supercontinent Rodinia.
Liquid rock on the surface of the Earth.
Mineral group in which the carbonate ion, CO3-2, is the building block. This can also refer to the rocks that are made from these minerals, namely limestone and dolomite (dolostone).
A group of chain silicate minerals that form needlelike or prismatic crystals. Can be many colors but the most common form, hornblende, is dark brown to black. Has oblique cleavages at 54° and 126°. Common in many igneous rocks and some metamorphic rocks.
The layers of igneous, sedimentary, and metamorphic rocks that form the continents. Continental crust is much thicker than oceanic crust. Continental crust is defined as having higher concentrations of very light elements like K, Na, and Ca, and is the lowest density rocky layer of Earth. Its average composition is similar to granite.
The innermost chemical layer of the Earth, made chiefly of iron and nickel. It has both liquid and solid components.
Place where two plates come together, casing subduction or collision.
The entire area which is related to land-sea interactions.
Metamorphism that occurs in subduction zones, typically lower temperature and higher pressure.
Name given to the subducting plate, where volatiles are driven out at depth, causing volcanism.
A process where an oceanic plate descends bellow a less dense plate, causing the removal of the plate from the surface. Subduction causes the largest earthquakes possible, as the subducting plate can lock as it goes down. Volcanism is also caused as the plate releases volatiles into the mantle, causing melting.
A metamorphic facies of low temperature, high pressure rocks, typified by the rock blueschist, a metamorphic rock containing a blue amphibole called glaucophane.
A very fine grained version of silica deposited with or without microfossils.
A property of solids in which a force applied to an object causes the object to fracture, break, or snap. Most rocks, at low temperatures, are brittle.
A type of breccia that forms in a brittle way within fault zones.
A property of a solid, such that when a force is applied, the solid flows, stretches, or bends along with the force, instead of cracking or breaking. For example, many plastics are ductile.
Fault-formed rock via ductile deformation, deeper within the Earth.
Component of the gravitational force which pushes material downslope.
Consisting of three end members: potassium feldspar (K-spar, KAlSi3O8), plagioclase with calcium (CaAl2Si2O8, called anorthite), and plagioclase with sodium (NaAlSi3O8, called albite). Commonly blocky, with two cleavages at ~90°. Plagioclase is typically more dull white and gray, and K-spar is more vibrant white, orange, or red.
Stress within an object that causes a side-to-side movement within an internal fabric or weakness.
Strong crystals that do not deform as easily under ductile deformation, and form lens-shaped porphyroblasts.
A stoney and/or metallic object from our solar system which was never incorporated into a planet and has fallen onto Earth. Meteorite is used for the rock on Earth, meteoroid for the object in space, and meteor as the object travels in Earth's atmosphere.
A large extraterrestrial object, such as a meteor or asteroid, that hits the surface of the Earth.
Metamorphism caused by bolide impacts.
A strain that occurs in a substance in which the item changes shape due to a stress.
Thin (less than 1 cm) beds of rock.
Minerals with the same composition and different crystal structures.
Place where lava is erupted at the surface.
A large crystal within an igneous rock. These can be seen within phaneritic and porphyritic rocks.
A piece of a rock that is caught up inside of another rock.
Igneous rock cooling, and thus forming, outside of the Earth, i.e. on the surface.