3 Fed and Fasted State
Learning Objectives
- Describe the effect of insulin, glucagon, epinephrine, and cortisol on metabolic processes in the liver, adipose, and skeletal muscle and how these hormones function to regulate blood glucose homeostasis.
- Determine the fuels utilized by the liver, red blood cells, adipose, skeletal muscle in the fed and fasted states and determine the pathway(s) providing this substrate.
- Differentiate between insulin sensitive and insulin insensitive tissues; identify the GLUT transporters common to specific tissues and their clinical relevance.
- Review the signaling mechanisms used by insulin, glucagon, cortisol, and epinephrine.
About this Chapter
One of the fundamental homeostatic responses is the regulation of blood glucose by alterations in flux through metabolic pathways. This regulation includes both dietary intake of fuels, tissue uptake and oxidation of fuels, and storage and release of fuels under necessary conditions. Most of these processes are controlled hormonally by insulin, glucagon, cortisol, and epinephrine. Ultimately, these pathways ensure that both ATP levels are sufficient for an organism to sustain cellular activities and that blood glucose is maintained in a narrow window. Both processes are balanced without exhausting either fuel and energy resources.
3.1 Fed and Fasted States
Glucose homeostasis is fundamental to the human body and regulated primarily by the levels of four major hormones:
- Insulin,
- Glucagon,
- Cortisol, and
- Epinephrine.
The ratios of these hormones in circulation will dictate the activity of specific metabolic pathways that control glucose homeostasis in a range of 80 mg/dL to 120 mg/dL. There are many other hormones (thyroid hormone, growth hormone, etc.) and adipokines (adiponectin, leptin, etc.) that can influence glucose homeostasis, as well as neural mechanisms that control higher level functions such as hunger and satiety. These will not be the focus of this section.
Fed state metabolism
In the fed state, or postprandial, elevated glucose levels trigger the release of insulin from the pancreas. As insulin levels rise, there is an increase in glucose uptake, oxidation, and storage in peripheral tissues as well as increases in other anabolic pathways.
Under these conditions, most tissues (liver, skeletal muscle, adipose, brain, and red blood cells) will increase glucose uptake and oxidation (table 3.1 and figure 3.1).
Each tissue will take up glucose in the fed state using one of the glucose transporters (GLUT) known to facilitate glucose transport across the plasma membrane. This family of proteins can be broadly categorized as insulin-independent and insulin-dependent transporters.
Tissue type | Fuel utilized in fed state | Primary glucose transporter |
---|---|---|
Liver | Glucose | GLUT2 (insulin-independent) |
Red blood cells | Glucose | GLUT1 (insulin-independent) |
Brain | Glucose | GLUT1 and GLUT3 (insulin-independent) |
Skeletal muscle | Glucose | GLUT4 (insulin-dependent) |
Adipose | Glucose | GLUT4 (insulin-dependent) |
Table 3.1: Summary table of fuels used in the fed state and uptake methods for important tissues.
Insulin-independent glucose uptake
The brain and red blood cells will always preferentially oxidize glucose regardless of hormone levels. Consequently, both tissues have a prevalence of GLUT1 transporters on the cell membrane. GLUT1 is present on the blood brain barrier, while GLUT3 is predominant on the brain. GLUT1 has a lower Km (higher affinity) for glucose, ensuring glucose transport to these important tissues. Likewise, glucose uptake in the liver is also insulin-independent and is facilitated by GLUT2 transporters. The pancreas also predominantly expresses GLUT1 and is able to take up glucose in this manner.
Red blood cell metabolism
The red blood cell lacks mitochondria, therefore it oxidizes glucose under both fed and fasted conditions. Glucose can be oxidized by:
- Glycolysis. Glucose is oxidized to pyruvate and converted to lactate, which will enter the Cori Cycle (section 4.1). (Lactate is returned to the liver and used as a substrate for gluconeogenesis.)
- Pentose phosphate pathway. Glucose is initially oxidized to ribulose 5-phosphate. In addition to the production of this 5-C sugar, more importantly NADPH is produced, which is needed as a reducing agent in the red blood cell (section 7.1).
Brain metabolism
The brain will preferentially oxidize glucose under most conditions with the exception of starvation states.
Liver metabolism
In the liver, glucose is taken up in an insulin-independent manner, and the activity of the following processes increased in the fed state are summarized in figure 3.1 and tables 3.1 and 3.2.
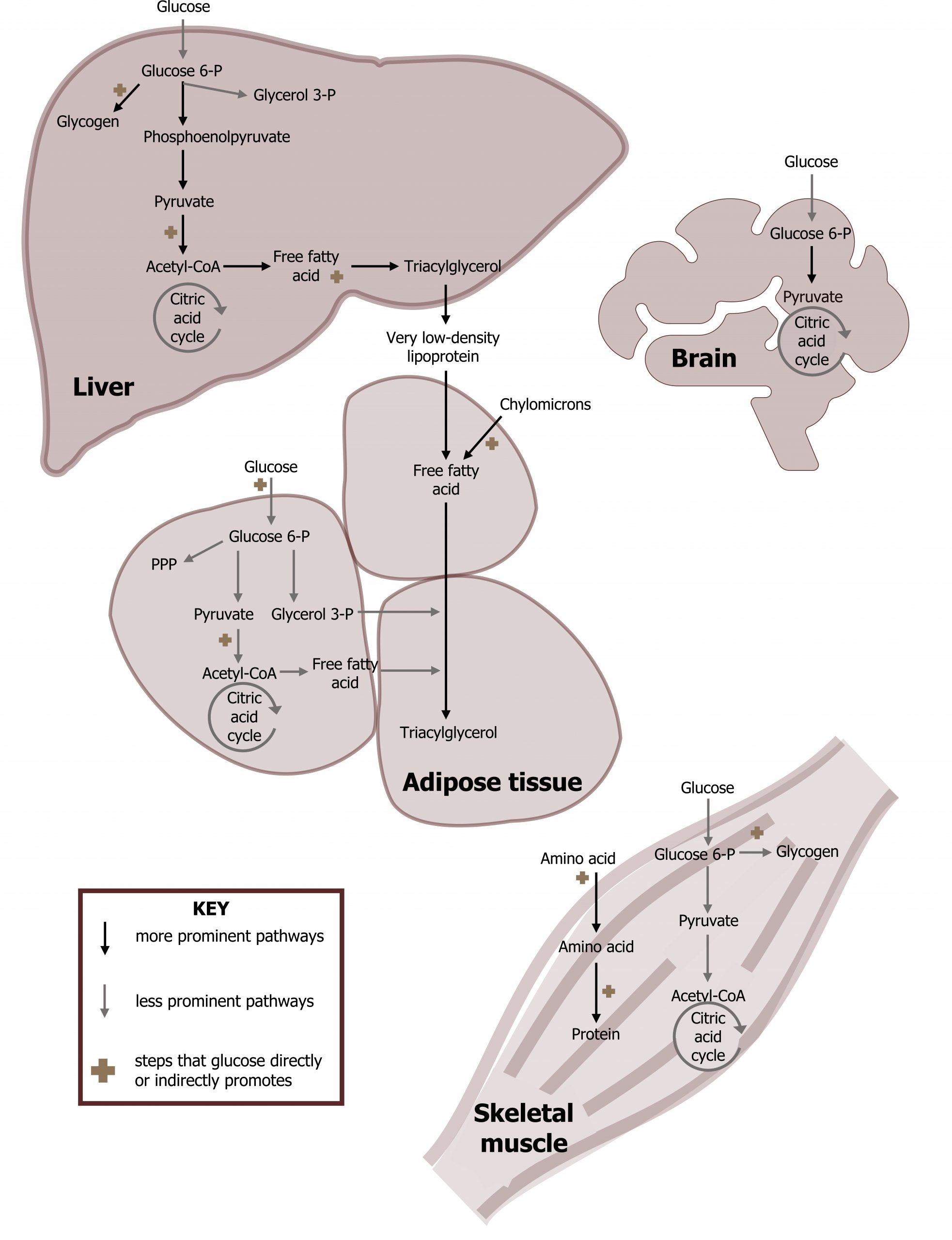
- Glycolysis. Glucose will be oxidized to pyruvate, which can enter the TCA cycle after conversion to acetyl-CoA (section 4.1).
- Glycogen synthesis. Glucose 6-phosphate is used to synthesize glycogen (section 4.5).
- Pentose phosphate pathway. Glucose 6-phosphate can be used to generate five-carbon sugars and NADPH (section 7.1).
- Tricarboxylic acid cycle. The TCA cycle will oxidize acetyl-CoA to generate NADH, FADH2, and GTP. The acetyl-CoA that enters the cycle is fully oxidized and released as CO2 (section 4.2).
- Cholesterol synthesis. Acetyl-CoA transported to the cytosol is used to synthesize cholesterol, which can be used for many cellular processes (section 6.1).
- Fatty acid synthesis. Excess citrate from the TCA cycle is shuttled out of the mitochondria and cleaved into oxaloacetate (OAA) and acetyl-CoA. The cytosolic acetyl-CoA can be used for fatty acid synthesis or cholesterol synthesis (section 4.4). Ultimately fatty acid synthesis leads to VLDL synthesis and secretion.
- Urea cycle. Depending on diet and translation needs, excess amino acids will be deaminated through transamination reactions, and the nitrogen will enter the urea cycle as aspartate or free ammonia. Excess amino acids are not stored, rather the deaminated carbon skeletons can be stored as glycogen or triacylglycerol (section 5.3).
Insulin-dependent glucose uptake
In contrast, the skeletal muscle and adipose tissues require insulin for glucose uptake. GLUT4 is the primary glucose transporter on these tissues, and in the absence of insulin this transporter is predominantly bound to intracellular vesicles. When the cell receives a signal (via insulin binding the insulin receptor), this cell signaling event allows the GLUT4 containing vesicles to fuse with the plasma membrane where it will facilitate glucose uptake.
Skeletal muscle metabolism
The skeletal muscle will increase uptake of both amino acids and glucose under fed conditions.
- Glycolysis. Glucose will be oxidized to pyruvate, which can enter the TCA cycle after conversion to acetyl-CoA (section 4.1).
- Protein synthesis. Amino acids will be used for protein synthesis (anabolic metabolism).
- Glycogen synthesis. Glucose 1-phosphate can be converted to UDP-glucose and stored as glycogen (section 4.5).
Adipose metabolism
In the adipose tissue, glucose as well as dietary fat and cholesterol (transported as chylomicrons; section 6.2) are taken up by the adipose tissue. Glucose has several potential fates described below, while dietary fat is stored as triacylglycerol.
- Glycolysis. Glucose can be oxidized to pyruvate which can enter the TCA cycle after conversion to acetyl-CoA (section 4.1).
- Pentose phosphate pathway. Glucose will be oxidized to generate NADPH needed for fatty acid synthesis (section 7.1).
- TAG synthesis. Oxidation of glucose to glycerol 3-phosphate is needed for the synthesis of triacylglycerols (TAGs).
Pathway | Summary | Regulatory enzyme |
---|---|---|
Glycolysis | Glucose oxidation to pyruvate | Glucokinase/hexokinase Phosphofructokinase-1 Pyruvate kinase |
Glycogen synthesis | Fuel storage | Glycogen synthase |
Pentose pathway | NADPH and 5-C sugars production | Glucose 6-phosphate dehydrogenase |
Tricarboxylic acid cycle | Oxidizes pyruvate to generate NADH and FADH2 | Pyruvate dehydrogenase complex α-ketoglutarate dehydrogenase Isocitrate dehydrogenase |
Cholesterol synthesis | Produces steroid hormone precursor | HMG-CoA reductase |
Fatty acid synthesis | Produces free fatty acids and transported as VLDL for fuel storage in peripheral tissues | Acetyl-CoA carboxylase |
Urea cycle | Nitrogen disposal | Carbamoyl phosphate synthetase I |
Table 3.2: Summary of metabolism during the fed state.
Fasted state metabolism
Approximately two hours after a meal, the decrease in serum glucose levels will lead to decreased insulin production in the pancreas. At this point in fasted state metabolism, the insulin to glucagon ratio becomes less than 1 (insulin low; glucagon high) with an additional increase of cortisol and epinephrine. Under these conditions tissues will transition to utilizing alternative fuels for energy as a means of maintaining glucose homeostasis. Fasted state metabolism will have limited impact on the oxidation of glucose by the brain and red blood cells, but it will lead to an increase in fatty acid oxidation by both the skeletal muscle and the liver (figure 3.6). The fatty acids oxidized by these tissues are released through the process of epinephrine-mediated lipolysis from the adipose. In the fasted state, the liver will primarily release glucose using both gluconeogenesis and glycogenolysis for the maintenance of blood glucose.
Tissue type | Fuel utilized in the fasted state | Pathway providing the fuel |
---|---|---|
Liver | Fatty acids | Lipolysis in the adipose |
Red blood cells | Glucose | Hepatic glycogenolysis and gluconeogenesis |
Brain | Glucose | Hepatic glycogenolysis and gluconeogenesis |
Skeletal muscle | Fatty acids | Lipolysis in the adipose |
Table 3.3: Summary table of fuels used in the fasted state and the pathways providing the fuel source.
Liver metabolism
The primary role of the liver in the fasted state is to synthesize and release glucose. To facilitate this task, the liver will use circulating free fatty acids as the primary fuel source to generate energy (ATP) for these homeostatic processes. (These processes are summarized in figure 3.2 and tables 3.3 and 3.4)
- Glycogenolysis. Hepatic glycogenolysis provides glucose that is released into the bloodstream to maintain blood glucose and provide an oxidizable substrate for the brain and RBCs (section 4.5).
- Gluconeogenesis. This is an anabolic process that synthesizes glucose from lactate, amino acids, or glycerol. This process is heavily reliant on the ATP generated from β-oxidation. The glucose produced is released into the bloodstream to maintain blood glucose and provide an oxidizable substrate for the brain and RBCs (section 5.1).
- Fatty acid β-oxidation. This is the process by which free fatty acids are oxidized to produce acetyl-CoA, NADH, and FADH2. It is a high energy yielding process and is required to generate ATP in the fasted state (section 5.2).
- Ketogenesis. This process utilizes the acetyl-CoA produced through β-oxidation to produce β-hydroxybutyrate and acetoacetate. These ketone bodies can be oxidized by peripheral tissues; the liver cannot oxidize ketone bodies (section 5.2).
- Urea cycle. Cortisol initiated protein catabolism provides amino acids needed as a substrate for gluconeogenesis. In order to use the carbon skeletons (keto-acids), the amino acids must be deaminated and the ammonia is disposed of through the synthesis of urea; nitrogen will enter the urea cycle as aspartate or free ammonia (section 5.3).
Red blood cell metabolism
The red blood cell lacks mitochondria, therefore it oxidizes glucose under both fed and fasted conditions. The metabolism of this tissue remains largely unchanged.
Brain metabolism
The brain will oxidize glucose under most conditions with the exception of starvation states. Under normal fasting conditions, although ketones will be synthesized, the brain will not transition to utilizing them as a predominant source of fuel until extended fasting has occurred (days).
Skeletal muscle metabolism
The skeletal muscle will increase uptake of fatty acids and ketones.
- Fatty acid β-oxidation. This is the process by which free fatty acids are oxidized to produce acetyl-CoA, NADH, and FADH2 (section 5.2).
- Oxidation of ketones. Ketone bodies taken up by the skeletal muscle can be reconverted to acetyl-CoA and oxidized in the TCA cycle (section 5.2).
- Protein catabolism. Cortisol-mediated protein catabolism is also active and supplies amino acids for gluconeogenesis in the liver.
Adipose metabolism
The most important process in the adipose tissue during the fasted state is lipolysis.
- Lipolysis. This process will release fatty acids from stored triacylglycerols and provides an oxidizable substrate for the skeletal muscle and liver (section 5.2).
Pathway | Summary | Regulatory enzyme |
---|---|---|
Glycogenolysis | Provides glucose for maintenance of blood glucose | Glycogen phosphorylase |
Gluconeogenesis | Provides glucose for maintenance of blood glucose | Glucose 6-phosphatase Fructose 1,6-biphosphatase PEPCK/pyruvate carboxylase |
Lipolysis | Releases free fatty acids from adipose | Hormone sensitive lipase |
Fatty acid β-oxidation | Generates ATP in the fasted state | Carnitine palmitoyltransferase |
Ketogenesis | Generates ketone bodies | Driven by substrate availability HMG-CoA synthase |
Urea cycle | Nitrogen disposal | Carbamoyl phosphate synthetase I |
Table 3.4: Summary of metabolism during the fasted state.
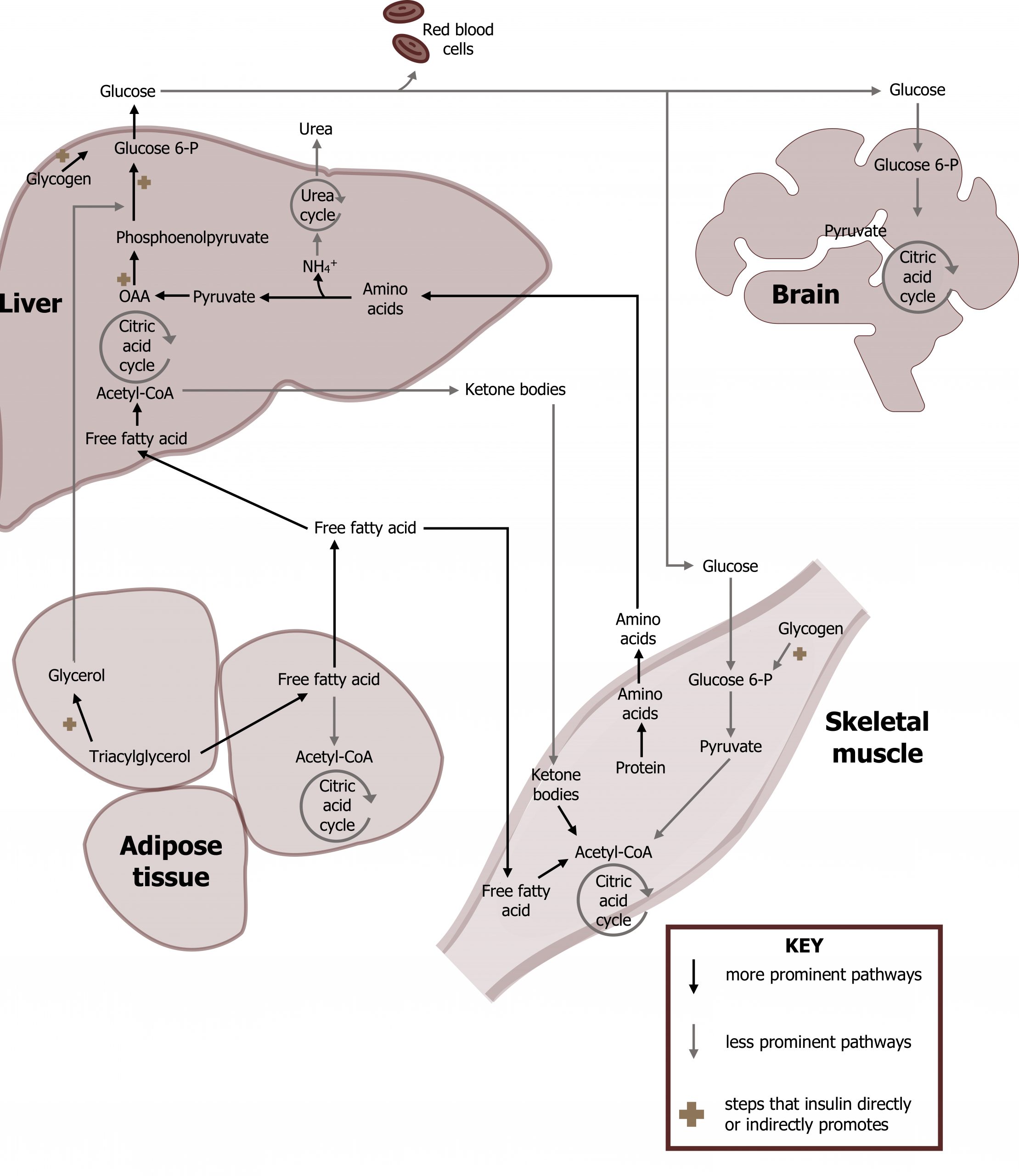
3.1 References and resources
Text
Ferrier, D. R., ed. Lippincott Illustrated Reviews: Biochemistry, 7th ed. Philadelphia: Wolters Kluwer Health/Lippincott Williams & Wilkins, 2017, Chapter 24: Fed Fast Cycle.
Le, T., and V. Bhushan. First Aid for the USMLE Step 1, 29th ed. New York: McGraw Hill Education, 2018, 91, 324–325
.
Lieberman, M., A. and Peet, eds. Marks’ Basic Medical Biochemistry: A Clinical Approach, 5th ed. Philadelphia: Wolters Kluwer Health/Lippincott Williams & Wilkins, 2018, Chapter 2: The Fed or Absorptive State, Chapter 3: The Fasted State.
Figures
Arm muscles anatomical. Public domain. From wpclipart.
Gregory D, Marshall D, Fat cells. CC BY 4.0. From Welcome Collection.
Grey, Kindred, Figure 3.1: Overview of fed state metabolism. 2021. CC BY 4.0. Added Liver by Liam Mitchell from the Noun Project, Brain by Maxicons from the Noun Project, and Muscle by Laymik from the Noun Project.
Grey, Kindred, Figure 3.2: Overview of fasted state metabolism. 2021. CC BY 4.0. Added Liver by Liam Mitchell from Noun Project, Brain by Maxicons from Noun Project, Muscle by Laymik from Noun Project, red blood cells by Lucas Helle from Noun Project.
Häggström M, Liver (transparent). Public domain. From Wikimedia Commons.
LadyofHats, Osmotic pressure on blood cells diagram. Public domain. From Wikimedia Commons.
_DJ_, Human brain on white background. 2005. CC BY-SA 2.0. From Flickr.