Chapter 1: Botany
Chapter Contents:
Horticulturists work with a wide array of plants, both as garden friend or foe. This range of different plants brings an enjoyable diversity to the garden and landscape, yet on close observation many similarities become evident. These similarities in basic plant structures and functions, along with the environmental factors that affect plant growth, are the basis of this chapter. The information provided in this chapter will primarily focus on the higher flowering plants because they are most significant in the garden and landscape. This chapter will cover terminology to help enhance your understanding of botanical references in the future and to gain a perspective on the practices discussed in later chapters.
Hundreds of millions of years of evolution have produced an amazingly diverse and complex array of plants. Land plants are primarily divided into two groups: gymnosperms and angiosperms. Angiosperms are flowering plants that produce seeds enclosed in a fruit. There are over 300,000 species of angiosperms distributed all over the world. The gymnosperms, numbering around 700 species, are primarily the evergreen species of the temperate zones. They produce naked seeds, which are usually borne in cones. Gymnosperms generally also have narrow or needle-like leaves, while angiosperms usually have broad leaves.
Angiosperms are first subdivided into two major subclasses based on their vascular (or vein) arrangement. The dicots include most of the broadleaf herbs, shrubs, and trees. Monocots include such orders as lilies, palms, and grasses.
Plant Cell Structure and Function
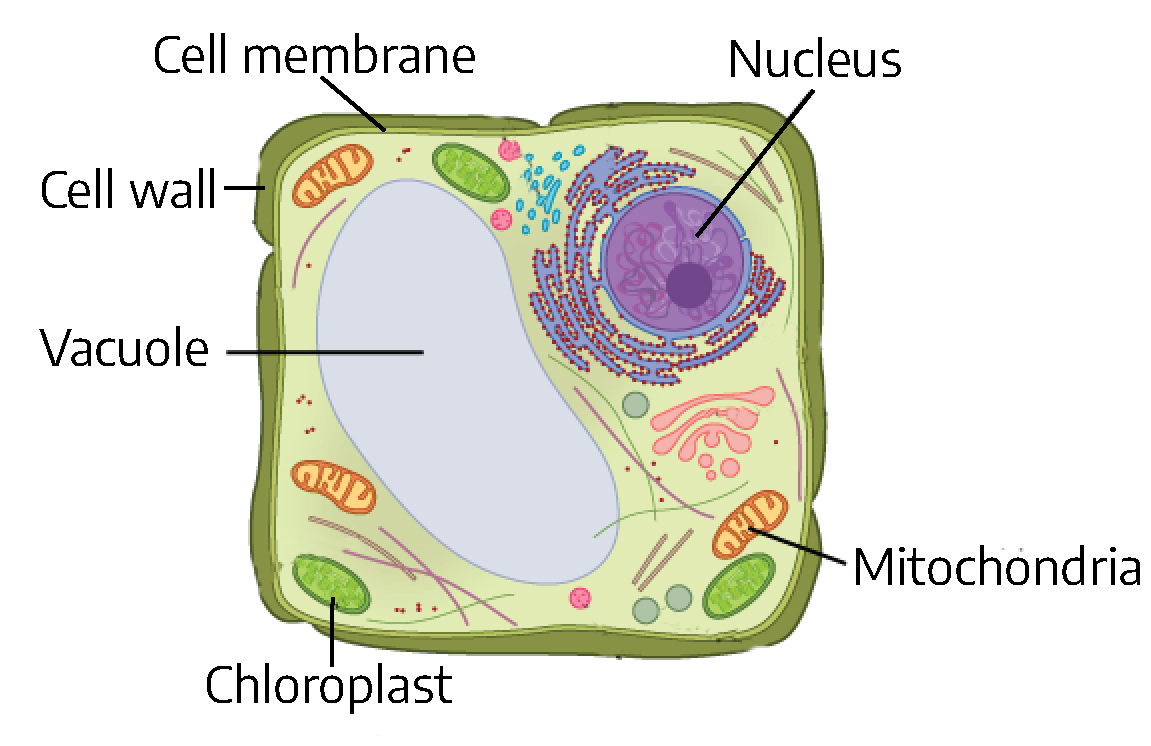
Cells are the structural and functional units of life. Large organisms are made up of trillions of cells, while small organisms may be composed of only a single cell. Both plants and animals are made of cells, though plant and animal cells are somewhat different.
Plant cells consist of a cell wall containing cellulose, a chemical compound. Inside the cell wall, a plant cell has some of the same features as animal cells: a cell membrane, mitochondria (which are responsible for respiration, or energy production), a nucleus (which contains the genetic information for the organism and controls the activities of the cell), and numerous other organelles necessary to carry out the mechanisms of life. Plant cells also develop one (or more) large liquid-filled cavity called a vacuole.
Plant cells have special structures called chloroplasts. Chloroplasts are the sites of photosynthesis and contain chlorophylls and carotenoid pigments. Chlorophyll is responsible for the green color of plants. Carotenoids are yellow and orange pigments that are masked by the more numerous chlorophyll pigments in green leaves. Chloroplasts are found only in plants and green algae (Evert and Eichhorn, 2012).
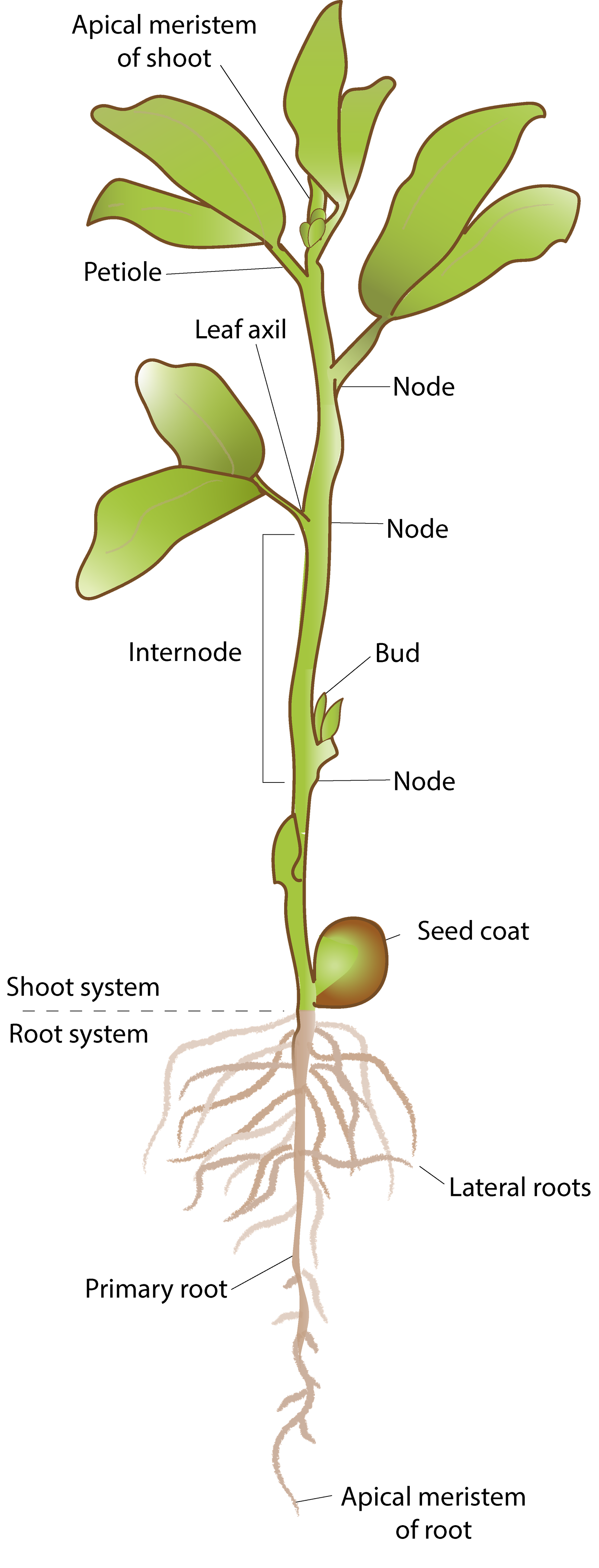
Plant cells grow and divide in different directions, creating all the structures of the plant like roots, stems, leaves, and more.
Anatomy: Plant Parts and Functions
Stem Anatomy
Stems are structures that support buds and leaves and serve as conduits for water, minerals, and sugars. The three major internal parts of a stem are the xylem, phloem, and cambium. The xylem and phloem are the major components of a plant’s vascular system. The vascular system transports food, water, and minerals and offers support for the plant. Xylem tubes conduct water and minerals to the leaves, while phloem tubes conduct sugars and other metabolic products away from the leaves.
The xylem and phloem make up the vascular system of the stem and are arranged in distinct strands called vascular bundles that run the length of the stem. When viewed in cross section, the vascular bundles of dicot stems are arranged in a ring. In plants with stems that live for more than one year, these individual bundles grow together (producing growth rings). In monocot stems, the vascular bundles are randomly scattered throughout the stem.
The difference in the vascular system of the two groups is of practical interest to the horticulturist because certain herbicides are specific to either monocots or dicots. An example is 2,4-D, an herbicide that only kills dicots. In contrast, dicots may be more readily grafted as it is easier to align the vascular rings of the two stem pieces compared to the scattered bundles in monocots.
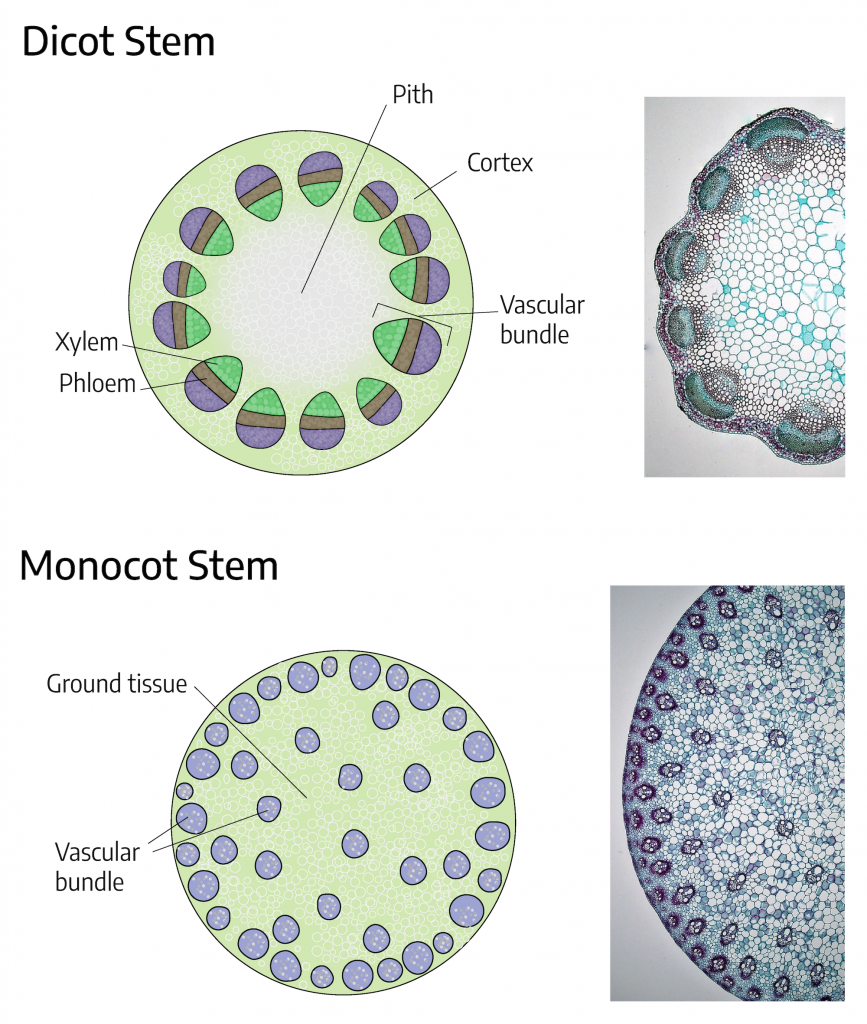
A part of the stem where a bud is located is called a node. This is where leaves are attached to the stem, and buds are located in these leaf axils (angle between stem and bud/leaf).
The stem section between nodes is called the internode. The length of an internode may depend on many factors. Internode length varies with the season. Growth produced early in the season has the greatest internode length; length decreases as the growing season nears its end. Decreasing fertility will decrease internode length. Too little light will result in a long internode, causing a spindly stem. This situation is known as stretch or etiolation. Vigorously growing plants tend to have greater internode lengths than less vigorous plants. Internode length will also vary with competition from surrounding stems or developing fruit. If the energy for a stem has to be divided among three or four stems, or if the energy is diverted into fruit growth, internode length will be shortened.
A bud is a small package of partially preformed tissue which becomes leaves/stems or flowers. In some cases, buds contain partially preformed flower tissue (flower bud), and usually have a different appearance from a vegetative bud, a bud that contains partially preformed leaf and stem tissue. Some buds contain both floral and vegetative tissues.
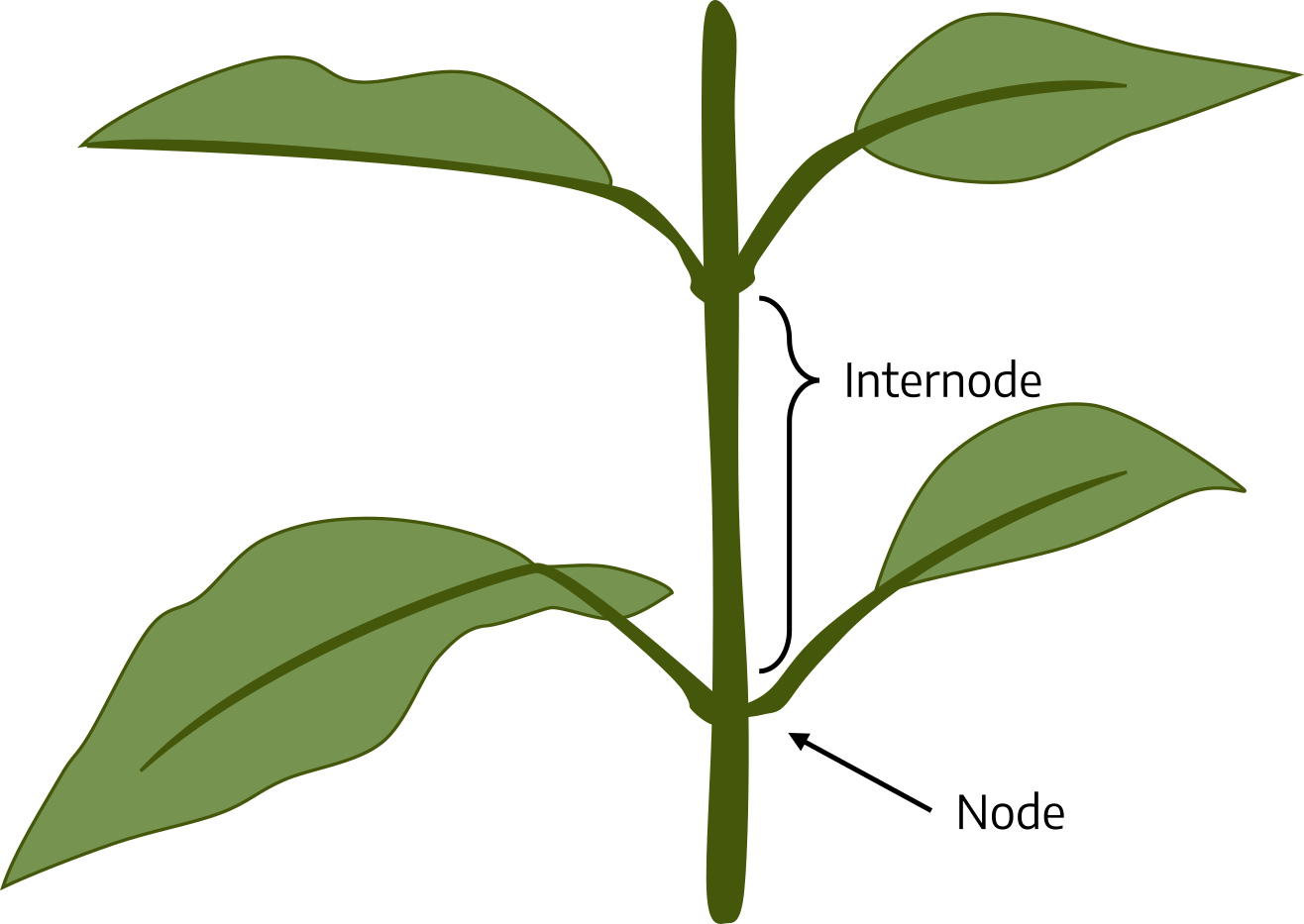
Modified stems
The presence of leaves (regular or modified) or buds distinguishes a stem. Although typical stems are above-ground trunks and branches with great distances between leaves and buds, there are modified stems that can be found above ground and below ground. The above-ground modified stems are crowns, stolons, and spurs; and the below-ground stems are bulbs, corms, rhizomes, and tubers.
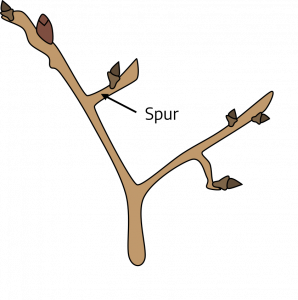
Above-ground stems
Spurs are short, stubby, side stems that arise from the main stem. They are common on such fruit trees as pears, apples, and cherries, and are capable of bearing fruit. If severe pruning is done close to fruit-bearing spurs, the spurs can revert to a long, nonfruiting stem.
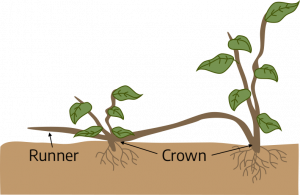
Crowns (seen in strawberries, dandelions, and African violets) are another type of compressed stem having leaves and flowers on short internodes. A crown is a region of compressed stem tissue from which new shoots are produced, generally found near the surface of the soil. Crowns are located at soil level so that roots support them upright and the central growing point is never covered with soil. Many herbaceous perennials, such as Shasta daisy, also develop crowns that enlarge with branching over successive years. These crowns persist over winter with buds that develop into elongated aerial stems during the growing season. A stolon is a horizontal stem that is fleshy or semi-woody and lies along the top of the ground. The spider plant has stolons. A runner is a type of stolon. It is a specialized stem that grows on the soil surface and forms a new plant at one or more of its nodes.
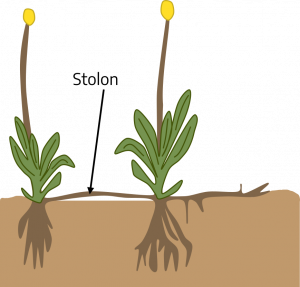
Strawberry runners are examples of stolons. Remember, all stems have nodes and buds or leaves. The leaves on strawberry runners are small, but are located at the nodes, which are easy to see. The nodes on the runner are the points where roots begin to form.
Below-ground stems, such as the potato tuber, the tulip bulb, gladiolus corm, and the iris rhizome, store food for the plant.
Below-ground stems
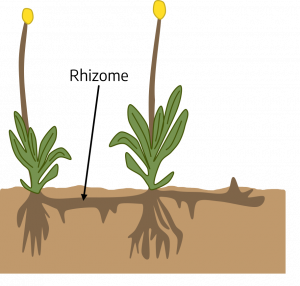
Rhizomes are similar to stolons but grow underground. Some rhizomes are compressed and fleshy, such as those of iris; they can also be slender with elongated internodes, such as bentgrass. Bermudagrass is both an effective lawn grass and a hated weed principally because of the spreading capability of its rhizomes.
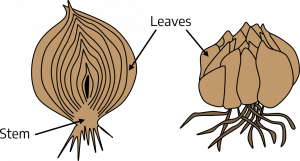
Tulips, lilies, daffodils, and onions are plants that produce bulbs — shortened, compressed, underground stems surrounded by fleshy scales (leaves) that envelop a central bud located at the tip of the stem. If you cut through the center of a tulip or daffodil bulb, you can see major plant parts within the bulb. Many bulbs require a period of low-temperature exposure before they begin to send up the new shoot. Both the temperature and length of this treatment are of critical importance to commercial growers who force bulbs for holidays.
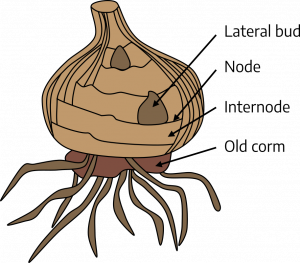
Corms are not the same as bulbs. They have shapes similar to bulbs, but do not contain fleshy scales. A corm is a solid, swollen stem whose scales have been reduced to a dry, leaf-like covering. Examples of corms include gladiolus and crocus.
A tuber is an enlarged portion of an underground stem. The tuber, like any other stem, has nodes that produce buds. The eyes of a potato are actually the nodes on the stem. Each eye contains a cluster of buds.
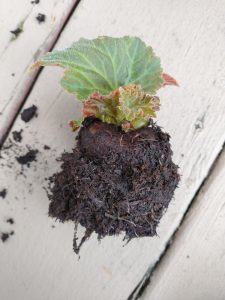
Some plants produce a modified stem referred to as a tuberous stem. Examples are tuberous begonia and cyclamen. The stem is shortened, flattened, enlarged, and underground. Buds and shoots arise from the crown, and fibrous roots are found on the bottom of the tuberous stem.
In addition, some plants, such as dahlia and sweet potato, produce an underground storage organ called a tuberous root, which is often confused with a bulb or tuber. However, these are roots, not stems, and have neither nodes nor internodes.
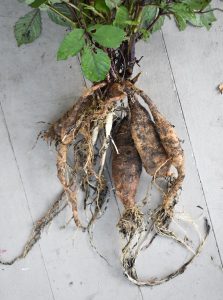
It may sometimes be difficult to distinguish between roots and stems, but one sure way is to look for the presence of nodes with their leaves and buds. Stems have nodes; roots do not.
Types of stems
A shoot is a young stem with leaves present. A twig is a stem that is less than one year old and has no leaves since it is still in the winter-dormant stage. A branch is a stem that is more than one year old and typically has lateral stems. A trunk is a main stem of a woody plant. Most trees have a single trunk.
Trees are perennial woody plants, usually with one main trunk and usually more than 12 feet tall at maturity.
Shrubs are perennial woody plants that have one or several main stems, and usually are less than 12 feet tall at maturity. The distinction between a small tree and large shrub is blurry, and often botanists will describe these plants as small trees or large shrubs.
A vine is a plant that develops long, trailing stems that grow along the ground unless they are supported by another plant or structure. Some twining vines circle their support clockwise (hops or honeysuckle), while others circle counter-clockwise (e.g., pole beans or Dutchman’s pipe vine). Clinging vines are supported by aerial roots (e.g.,English ivy or poison ivy). A tendril is a modified plant part (leaf, stem, or flower, depending on the plant) that encircles the supporting object (e.g., cucumber, gourds, grapes, and passionflowers). Some tendrils have adhesive tips (e.g., Virginia creeper and Japanese creeper).
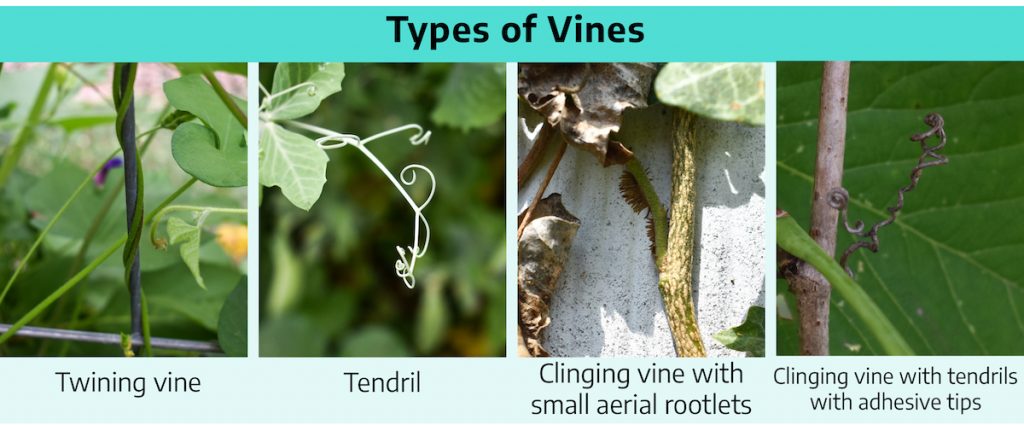
Texture and growth of stems
Woody stems contain relatively large amounts of hardened xylem tissue in the central core and are typical of most fruit trees, ornamental trees, and shrubs.
A cane is a stem that has a relatively large pith (the central, strength-giving tissue of stem) and usually lives only one or two years. Examples of plants with canes include rose, grape, blackberry, and raspberry.
Herbaceous or succulent stems contain only small amounts of xylem tissue and usually live for only one growing season. If the plant is perennial, it will develop new shoots from a crown or underground part. An example of a plant with herbaceous stems is mayapple (Podophyllum peltatum), a native perennial that grows back each year from underground roots.
The edible portion of cultivated plants such as asparagus and kohlrabi is an enlarged succulent stem. The edible parts of broccoli are composed of stem tissue, flower buds, and a few small leaves. The edible part of potato is a fleshy, underground tuber. Although the name suggests otherwise, the edible part of the cauliflower is immature inflorescence (flowers) and flower stalk.
Plant life cycles
Plants are classified by the number of growing seasons required to complete a life cycle.
- Annuals pass through their entire life cycle from seed germination to seed production in one growing season, then die.
- Biennials are plants that start from seeds and produce vegetative structures and food storage organs the first season. In most biennials, during the first winter a hardy evergreen rosette of basal leaves persists. During the second season, flowers, fruit, and seeds develop to complete the life cycle. The plant then dies. Carrot, beet, cabbage, and celery are biennial plants. Hollyhock, Canterbury Bells, and Sweet William are biennials commonly grown for their attractive flowers.
- Plants that typically develop as biennials may, in some cases, complete the cycle of growth from seed germination to seed production in only one growing season. This situation occurs when drought, variations in temperature, or other climatic conditions cause the plant to physiologically pass through the equivalent of two growing seasons in a single season.
- Perennial plants live for many years, and after reaching maturity, may produce flowers and seeds each year, though many only flower every few years. Perennials are classified as herbaceous if the top dies back to the ground each winter and new stems grow from the roots each spring. If significant xylem develops in the stem and the top persists, as in shrubs or trees, then they are classified as woody plants.
Leaf Anatomy
Parts of a leaf
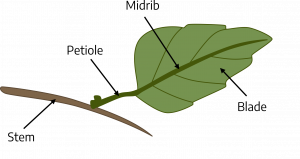
Leaves are the principal site of photosynthesis (food manufacturing) in plants. This process requires light and water from the plant’s vascular system, and carbon dioxide from the air. The petiole and leaf blade provide good exposure to sunlight and air. Other structures in the leaf are involved in accomplishing photosynthesis and protecting the soft leaf tissues from desiccation (drying out).
The blade of a leaf is the expanded, thin structure on either side of the midrib. The blade is usually the largest and most conspicuous part of a leaf. Conifers (gymnosperm class) have a different structure in that the leaf blade is a narrow angle needle. These needles occur singly or in clusters of two or more with their bases enclosed in a sheath. The petiole is the stalk that supports the leaf blade; it varies in length and may be lacking entirely in some cases where the leaf blade is described as sessile (attached directly by its base), as in conifers, grass, or zinnias.
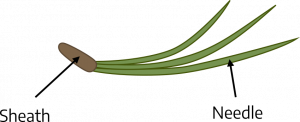
The leaf blade is composed of several layers. On both the top and bottom is a layer of thickened, tough cells called the epidermis. The primary function of the epidermis is protection of leaf tissue. The way the cells in the epidermis are arranged determines the texture of the leaf surface. Some leaves have hairs that are an extension of certain cells of the epidermis. The African violet has so many hairs that the leaf feels like velvet.
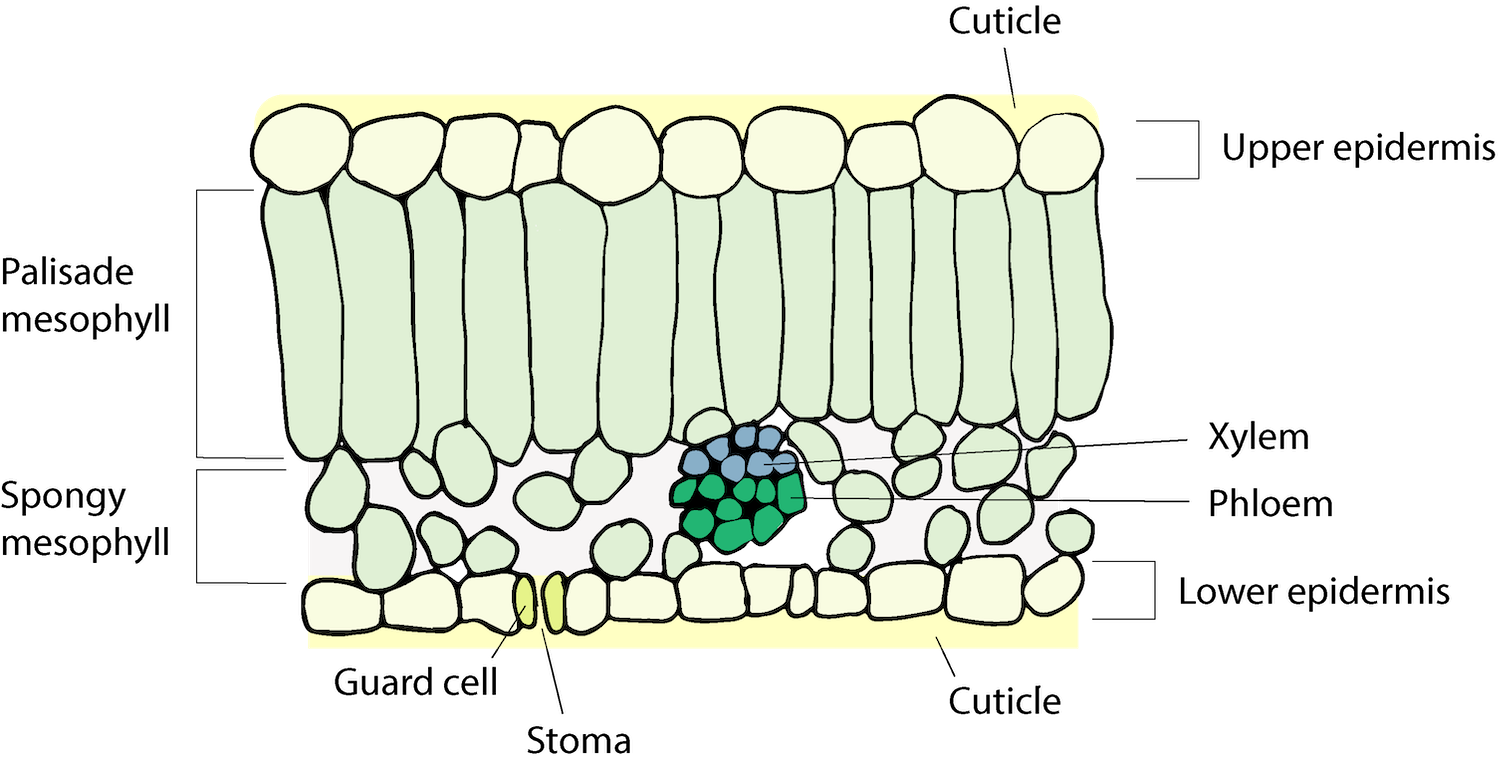
Part of the epidermis is the cuticle, which is composed of a waxy substance called cutin that protects the leaf from dehydration and prevents penetration of some diseases. The amount of cutin is a direct response to sunlight, increasing with increasing light intensity. For this reason, plants grown in the shade should be moved into full sunlight gradually over a period of a few weeks to allow the cutin layer to build and to protect the leaves from the shock of rapid water loss or sunscald. The waxy cutin also repels water and can shed pesticides if spreader-sticker agents or soaps are not used. This is the reason many pesticide manufacturers include some sort of spray additive to adhere to or penetrate the cutin layer.
Stomata (singular: stoma) are openings in leaves that allow passage of water and gasses into and out of the leaf. Guard cells are epidermal cells located around a stoma. They help regulate gas exchange by opening and closing in response to weather conditions.
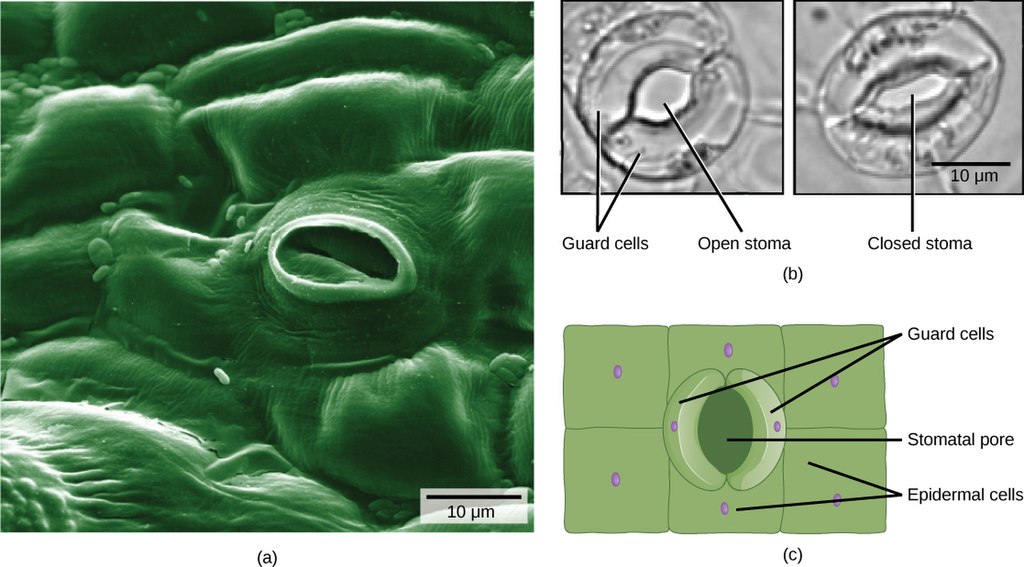
The middle layer of the leaf is the mesophyll and is located between the upper and lower epidermis. This is the layer where photosynthesis occurs. The mesophyll is divided into a dense upper layer called the palisade, and a spongy lower layer that contains a great deal of air space, called the parenchyma layer. The cells in these two layers contain chloroplasts which are the actual site of the photosynthetic process.
Types of leaves
A number of rather distinct types of leaves occur on plants. Leaves commonly referred to as foliage are the most common and conspicuous and, serve as the manufacturing centers where the photosynthetic activity of the plant occurs. Scale leaves, or cataphylls, are found on rhizomes and are the small, leathery, protective leaves that enclose and protect buds. Seed leaves, or cotyledons, are modified leaves that are found on the embryonic plant and commonly serve as storage organs. Spines as found on barberry and cactus, are specialized modified leaves that protect the plant. Storage leaves, as found on bulbous plants and succulents, serve as food storage organs. Other specialized leaves include bracts, which are often brightly colored. The showy structures on dogwoods and poinsettias are bracts, not petals.
The leaf blade is the principal edible part of several horticultural crops, including chive, collard, dandelion, endive, kale, leaf lettuce, mustard, parsley, spinach, and Swiss chard. The edible part of leek, onion, and Florence fennel is a cluster of fleshy leaf bases. The petiole of the leaf is the edible product in celery and rhubarb. In Brussels sprout, cabbage, and head lettuce, the leaves — in the form of a large, naked bud — are the edible product.
Leaves as a means of identifying plants
Leaves are useful in identifying species and varieties of horticultural plants. Characteristics of leaf composition, arrangement, and venation pattern can be readily recognized.
Composition:
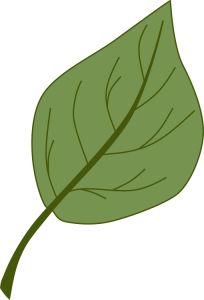
Simple leaves are those with a leaf blade that is a single continuous unit.
A compound leaf is composed of several separate leaflets arising from the same petiole. Some leaves may be doubly compound, having divisions of the leaflets. Compound leaves are further described by the palmate (as in palm of the hand) or pinnate (like a feather) arrangement of the leaflets.
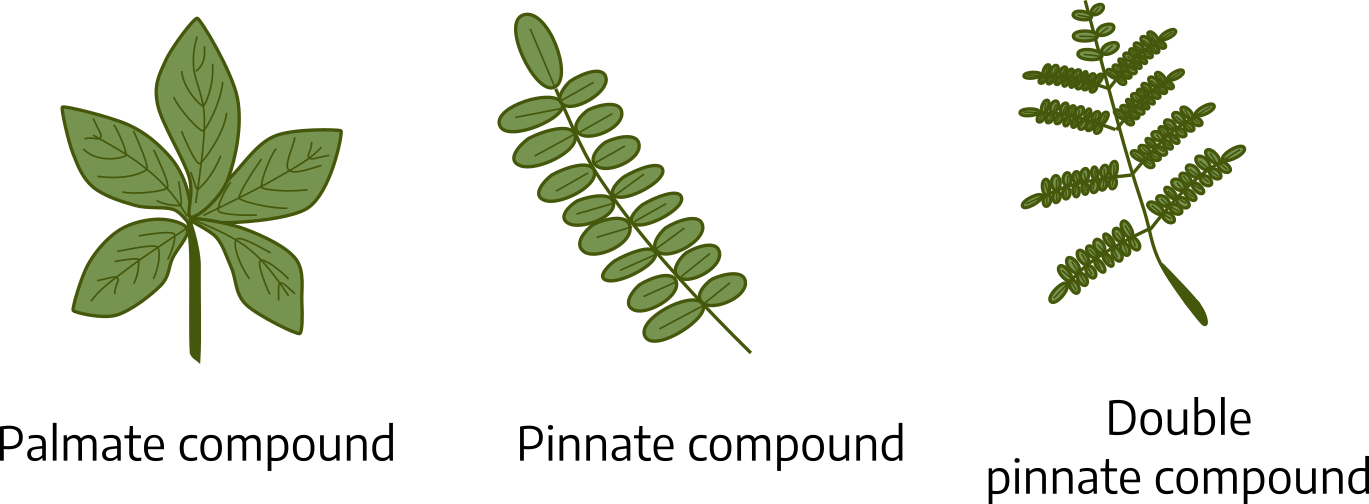
Deciding if a specimen is a compound leaf or a branch with several simple leaves can be difficult because petioles and young stems appear similar. However, remember that leaves attach to stems at a node, and there is a bud only at the node. The presence of a bud identifies where the leaf begins.
Leaf arrangement along a stem: In a rosulate leaf arrangement, the basal leaves form a rosette around the stem with extremely short nodes. Opposite leaves are positioned across the stem from each other, two leaves at each node. Alternate leaves are arranged in alternate steps along the stem with only one leaf at each node. Whorled leaves are arranged in circles along the stem.
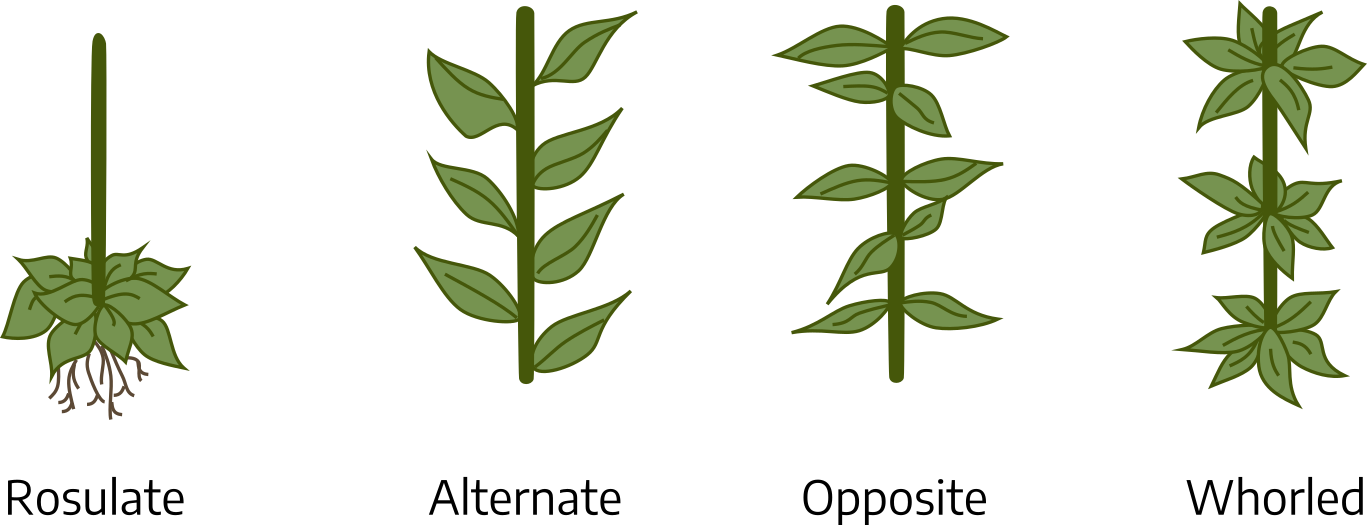
Venation of leaves
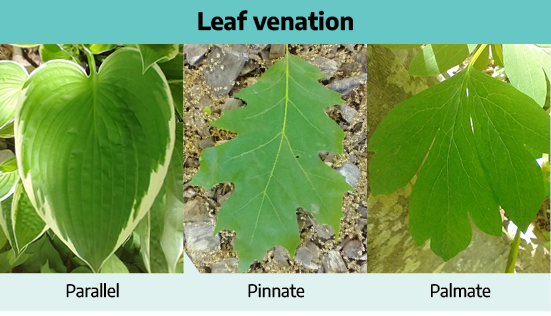
The vascular tissues from the stem extend as bundles through the petiole and spread out into the blade. The term venation refers to the pattern of vein distribution in the blade. Two principal types of venation are parallel-veined and net-veined.
Parallel-veined leaves are those with numerous veins that run essentially parallel to each other and are connected laterally by minute, straight veinlets. Possibly the most common type of parallel veining is that found in plants of the grass family, where the veins run from the base to the apex of the leaf. Another type of parallel venation is found in plants such as banana, calla, and pickerel-weed, where the parallel veins run laterally from the midrib. Parallel-veined leaves mainly occur on monocot plants.
Net-veined leaves,, also called reticulate-veined, have veins that branch from the main rib(s), then subdivide into finer veinlets which then unite in a complicated network. This system of enmeshed veins gives the leaf more resistance to tearing than most parallel-veined leaves. Net venation may be either pinnate or palmate. In pinnate venation, the veins extend laterally at an angle from the midrib to the edge, as in apple, cherry, and peach.
Palmate venation occurs in grape and maple leaves where the principal veins extend outward, like the ribs of a fan, from the petiole near the base of the leaf blade. Net-veined leaves occur on dicot plants..
Leaf shape
Common leaf blade shapes (found in leaves and leaflets):
- Acicular: Needle-like
- Subulate: Awl-shaped with tapering point
- Linear: Narrow, several times longer than wide; approximately the same width throughout
- Oblong: Leaf is 2-3x as long as it is wide and has parallel sides
- Elliptical: Two or three times longer than wide; tapering to an acute or rounded apex and base
- Ovate: Egg-shaped, basal portion wide; tapering toward the apex
- Obovate: Leaf is broadest above the middle and about 2x as long as wide
- Lanceolate: Longer than wide; tapering toward the apex and base
- Oblanceolate: Leaf is 3x longer than wide and broadest above the middle
- Spatulate: Generally narrow leaves widening to a round shape at the tip
- Reniform: Leaves wider than they are high
- Hastate: Arrowhead shaped leaves
- Deltoid: Triangular
- Cordate: Heart-shaped, broadly ovate; tapering to an acute apex, with the base turning in and forming a notch where the petiole is attached
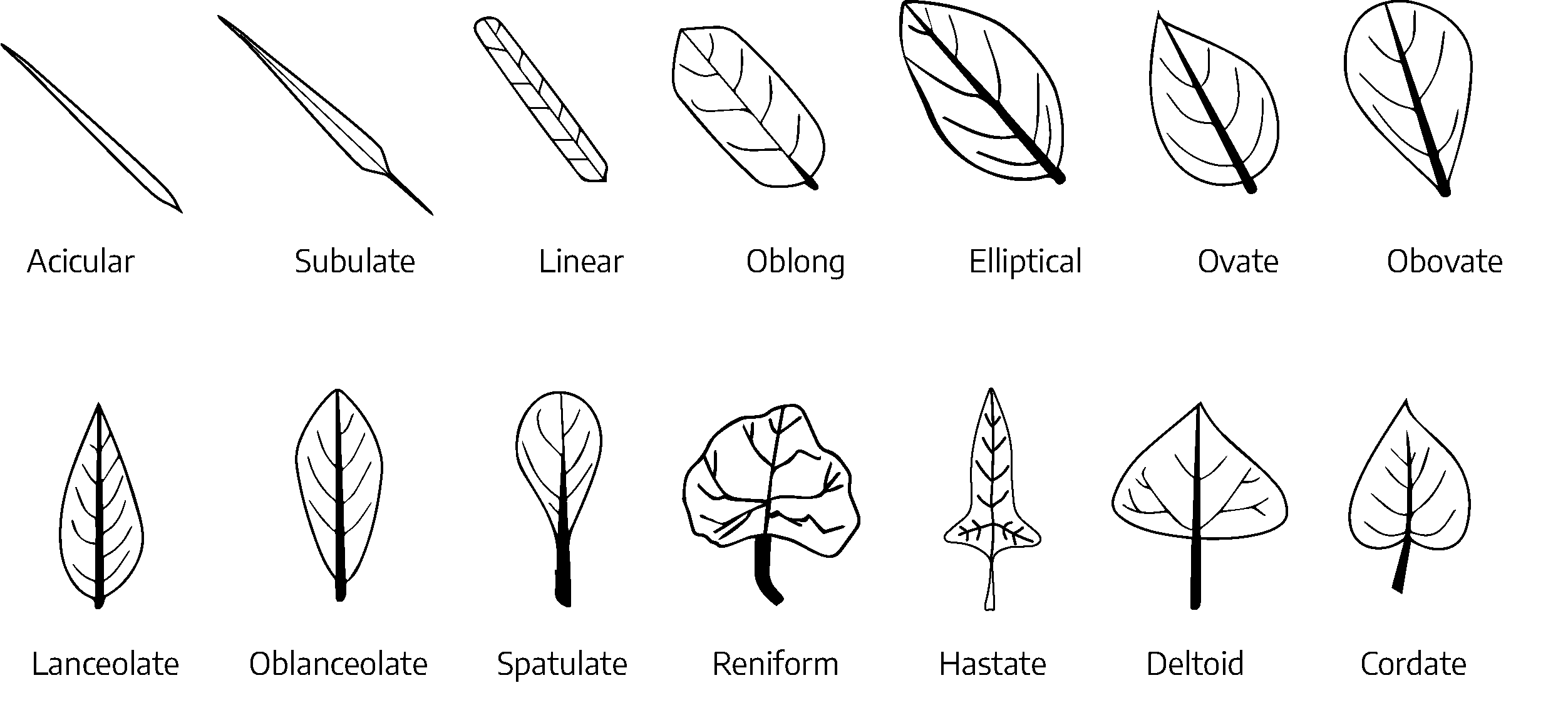
Leaf apex shapes:
- Acute: Ending in an acute angle with a sharp, but not acuminate, point
- Acuminate: Tapering to a long, narrow point
- Aristate: Has a bristle at the tip
- Cuspidate: Tip is sharp with two curves meeting at tip
- Mucronate: Tip ends in a small sharp point projecting from the midrib
- Obtuse: Tapering to a rounded edge
- Retuse: Rounded with a very shallow notch at apex
- Emarginate: Shallowly notched at the apex

Leaf base shapes:
- Cuneate: Wedge-shaped, gradually narrowed towards base
- Attenuate: Tapering with concave margins
- Obtuse: Blunt, forming a wide angle at the base
- Cordate: Rounded double lobes
- Auriculate: Ear-like appendages at the base
- Sagittate: Arrowhead-shaped, with two pointed lower lobes
- Truncate: Relatively square end

Leaf margins (studying leaf margins is especially useful in the identification of certain varieties of fruit plants):
- Entire: A smooth edge with no teeth or notches
- Sinuate: Having a pronounced sinuous or wavy margin
- Crenate: Having rounded teeth
- Dentate: Having teeth ending in an acute angle, pointing outward
- Serrate: Having small, sharp teeth pointing toward the apex
- Serrulate: Edges with smaller, more evenly-spaced serrations than a serrated leaf
- Double serrate: Small serrations on larger serrations
- Incised: Margin cut into sharp, deep, irregular teeth or incisions
- Lacerate: Irregular, appearing torn
- Pectinate: Prominent serrated teeth
- Ciliate: Fringed or fuzzy hairs along the margin
- Lobed: Incisions extend less than halfway to the midrib
- Cleft: Incisions extend more than halfway to the midrib
- Parted: Cut or dissected almost to the midrib
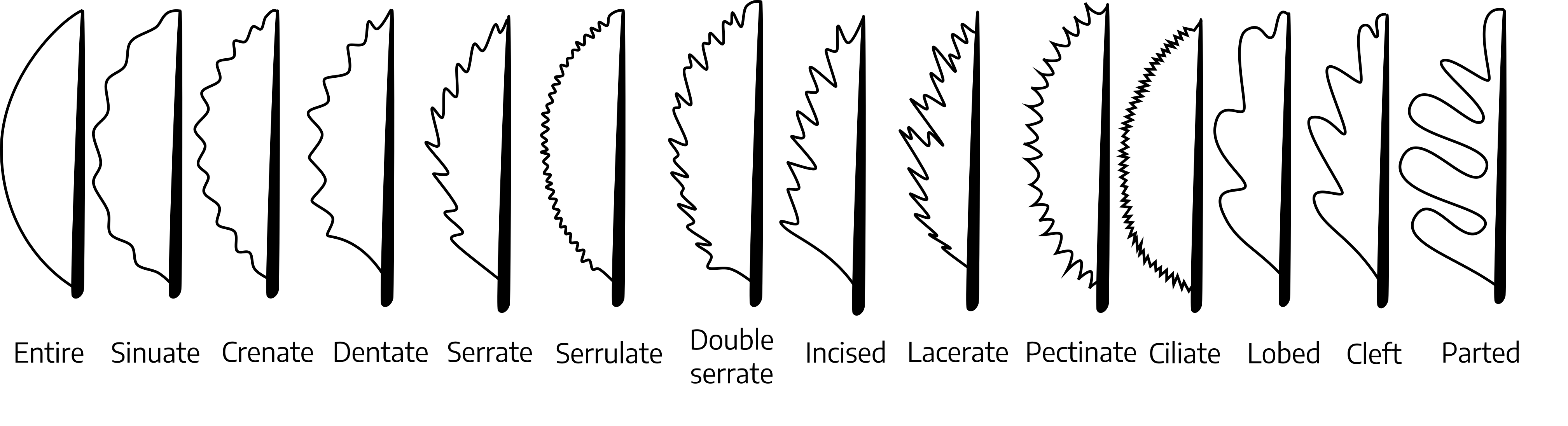
Bud Anatomy
A bud is an undeveloped shoot from which embryonic leaves or flower parts arise. The buds of trees and shrubs of the temperate zone typically develop a protective outer layer of small, leathery bud scales. Annual plants and herbaceous perennials have naked buds with outer leaves that are green and somewhat succulent.
Buds of many plants require exposure to a certain number of days below a critical temperature (a period of rest) before they will resume growth in the spring. This time period varies for different plants. The flower buds of forsythia require a relatively short rest period and will grow at the first sign of warm weather. Many peach varieties require from 700 to 1000 hours of temperatures below 45°F (7°C) before they will resume growth. During rest, dormant buds can withstand very low temperatures, but after the rest period is satisfied, buds become more susceptible to weather conditions and can be damaged easily by cold temperatures or frost.
A leaf bud is composed of a short stem with embryonic leaves, with bud primordia (early bud embryo) in the axils and at the apex. Such buds develop into leafy shoots. Leaf buds are often less plump and more pointed than flower buds.
A flower bud is composed of a short stem with embryonic flower parts. In some cases, the flower buds of plants that produce fruit crops of economic importance are called fruit buds. This terminology is objectionable because, although flowers have the potential for developing into fruit, this development may never occur because of adverse weather conditions, lack of pollination, or other unfavorable circumstances. The structure is a flower bud and should be so designated since it may never set fruit.
Types of buds
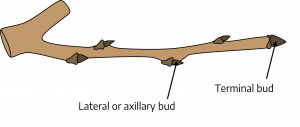
Buds are named for their location on the stem. Terminal buds are located at the apex of a stem. Lateral buds are borne on the sides of the stem. Most lateral buds arise in the leaf axils and are called axillary buds. In some instances, more than one bud is formed. Adventitious buds are those arising at sites other than in the terminal or axillary position. They may develop from the internode of the stem, at the edge of a leaf blade, from callus tissue at the cut end of a stem or root, or laterally from the roots of a plant.
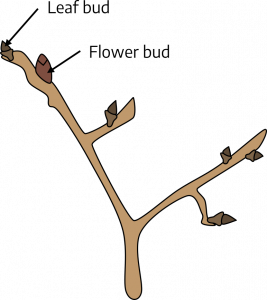
Enlarged buds or parts of buds form the edible portion of some horticultural crops. Cabbage and head lettuce are examples of unusually large terminal buds. Succulent axillary buds of Brussels sprouts become the edible part of this plant. In the case of globe artichoke, the fleshy basal portion of the bracts of the flower bud are eaten along with the solid stem portion of the bud. Broccoli is the most important horticultural plant having edible flower buds that are consumed. In this case, portions of the stem as well as small leaves associated with the flower buds are eaten.
Root Anatomy
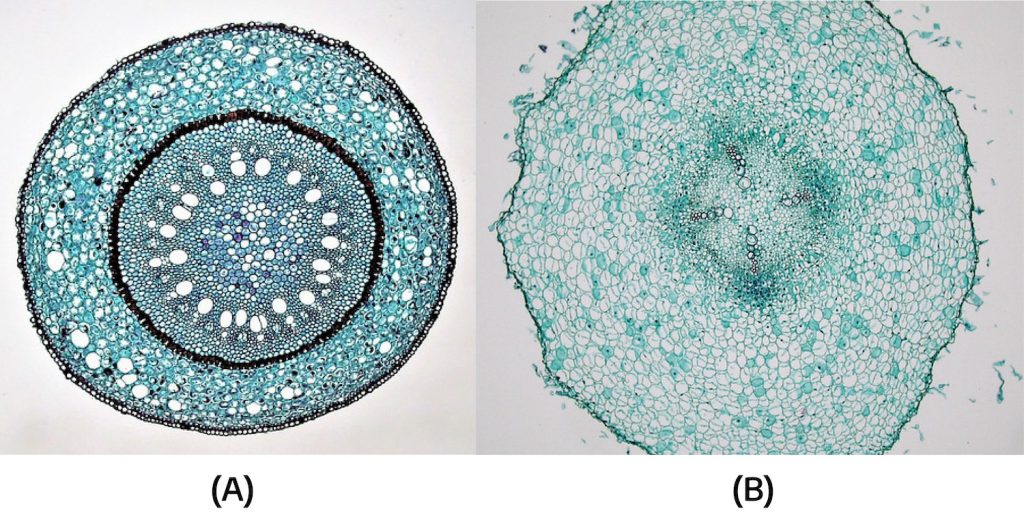
A thorough knowledge of the root system of plants is essential for understanding their growth, flowering, and fruiting responses. The structure and growth habits of roots have a pronounced effect on the size and vigor of the plant, method of propagation, adaptation to certain soil types, and response to cultural practices and irrigation. The roots of certain vegetable crops are important as food.
Roots typically originate from the lower portion of a plant or cutting. They possess a root cap, have no nodes, and never bear leaves or flowers directly. The principal functions of roots are to absorb nutrients and moisture, to anchor the plant in the soil, to furnish physical support for the stem, and to serve as food storage organs. In some plants, they may be used as a means of propagation.
Types of roots
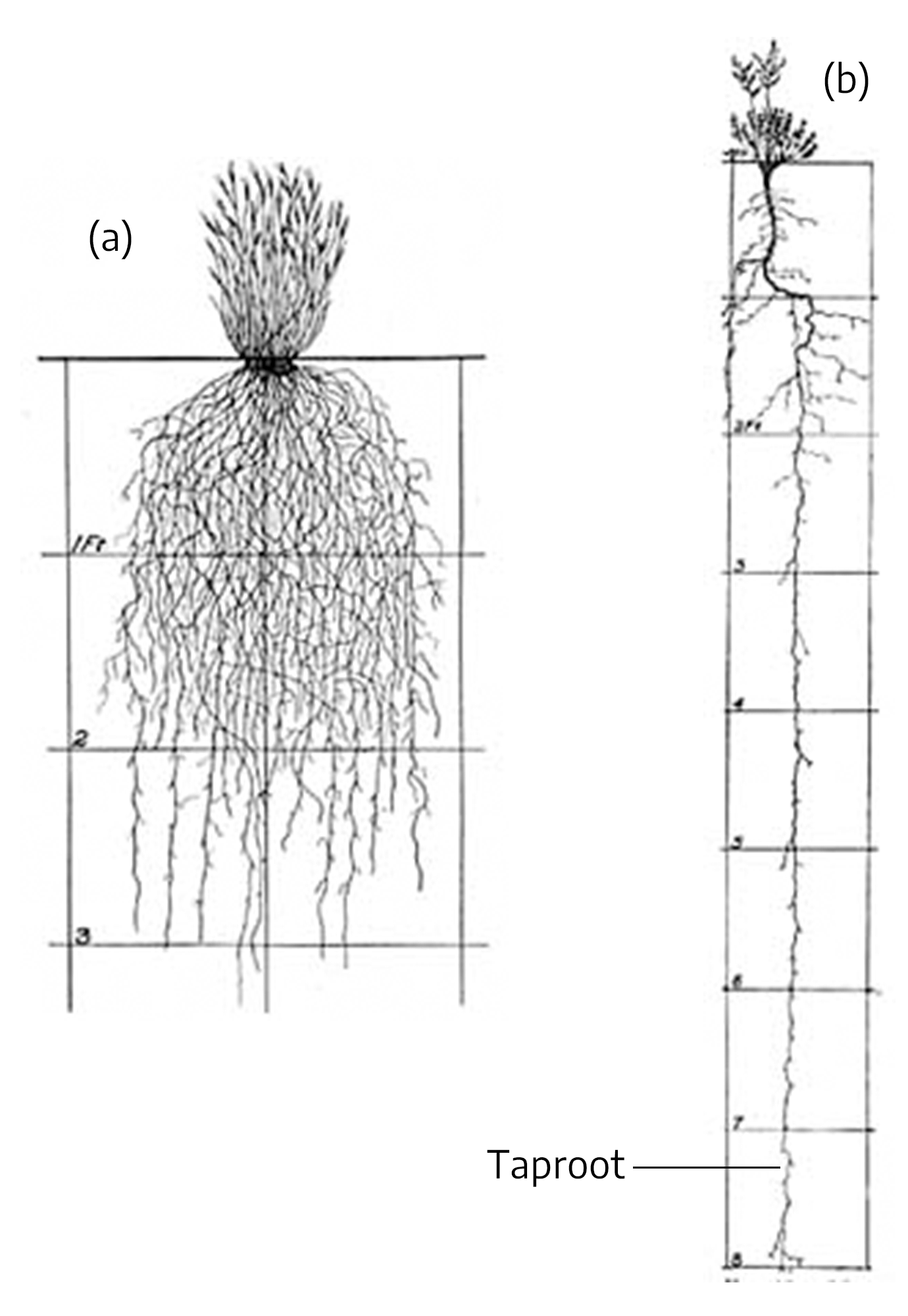
A primary (radicle) root originates at the lower end of the embryo of a seedling plant. A taproot is formed when the primary root continues to elongate downward into the soil and becomes the central and most important feature of the root system, with a somewhat limited amount of secondary branching. Some trees, especially nut trees like pecan, have a long taproot with very few lateral or fibrous roots. This makes them difficult to transplant and necessitates planting only in deep, well-drained soil.
A lateral, or secondary, root is a side or branch root that arises from another root.
A fibrous root system is one where the primary root ceases to elongate, leading to the development of numerous lateral roots which branch repeatedly and form the feeding root system of the plant. One factor that causes shrubs and dwarf trees to remain smaller than standard trees is the inactivity of the cambium tissue in the roots.
If plants that normally develop a taproot are undercut so that the taproot is severed early in the plant’s life, the root will lose its taproot characteristic and develop a fibrous root system. This is done commercially in nurseries so trees, which naturally have tap roots, will develop a compact, fibrous root system. This allows a higher rate of transplanting success.
The quantity and distribution of plant roots are very important because these two factors have a major influence on the absorption of moisture and nutrients. The depth and spread of the roots are dependent on the inherent growth characteristics of the plant and the texture and structure of the soil. Roots will penetrate much deeper in a loose, well-drained soil than in a heavy, poorly drained soil. A dense, compacted layer in the soil will restrict or stop root growth.
During early development, a seedling plant absorbs nutrients and moisture from the few inches of soil surrounding it. Therefore, the early growth of most horticultural crops that are seeded in rows benefits from band applications of fertilizer, placed several inches to each side and slightly below the seeds.
As plants become well established, the root system develops laterally and usually extends far beyond the spread of the branches. The greatest concentration of fibrous roots occurs in the top foot of soil, but significant numbers of laterals may grow downward from these roots to provide an effective absorption system several feet deep. Tuberous roots are modified lateral roots that are enlarged to function as a storage organ.
The enlarged root is the edible portion of several vegetable crops. The sweet potato is a tuberous root. Carrot, parsnip, salsify, and radish are elongated taproots.
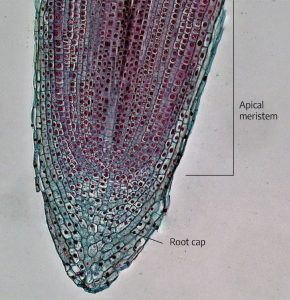
Parts of a root
Internally, there are three major parts of a root. The meristem is at the tip and manufactures new cells; it is an area of cell division and growth. Behind it is the zone of elongation, where cells increase in size through food and water absorption. These cells, by increasing in size, push the root tip through the soil. The third major root part is the maturation zone, where cells undergo changes to become specific tissues, such as epidermis, cortex, or vascular tissue. The epidermis is the outermost layer of cells surrounding the root. These cells are responsible for the absorption of water and minerals dissolved in water. Cortex cells are involved in the movement of water from the epidermis and in food storage. Vascular tissue is located in the center of the root and transports food and water.
Externally, there are two areas of importance. Root hairs are found along the main root and perform much of the actual work of water/nutrient absorption. The root cap is the outermost tip of the root and consists of cells that are sloughed off as the root grows through the soil. The root cap covers and protects the meristem.
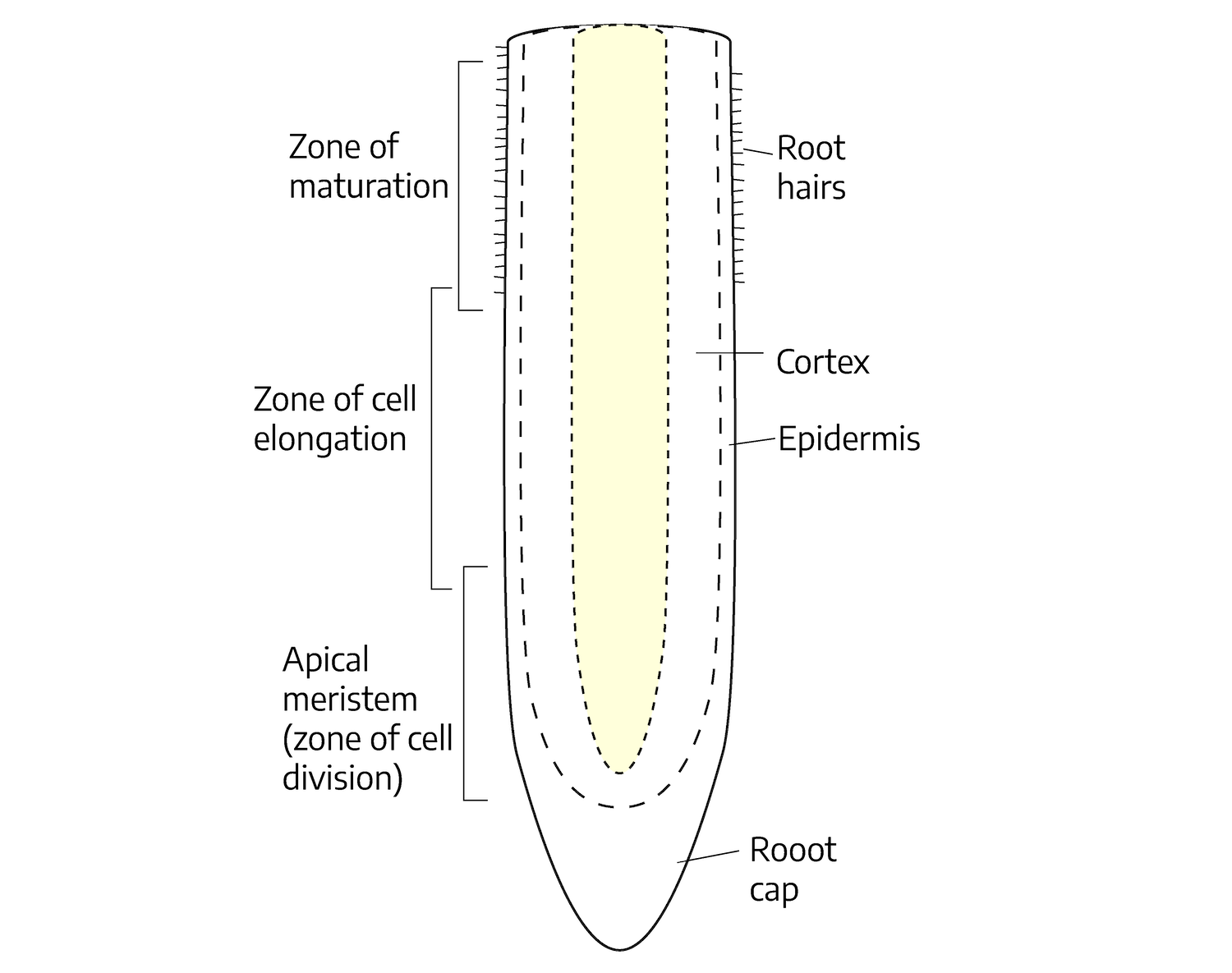
overlap with the zone of cell elongation, where cells grow and get longer, and with the zone of
maturation, where different root tissues (like the vascular system) mature at different rates and root hairs develop.
Flower Anatomy
The sole function of the flower, which is generally the showiest part of the plant, is sexual reproduction. Its attractiveness and fragrance have not evolved to please humans but to ensure the continuance of the plant species. Fragrance and color are devices to attract pollinators — insects that play an important role in the reproductive process.
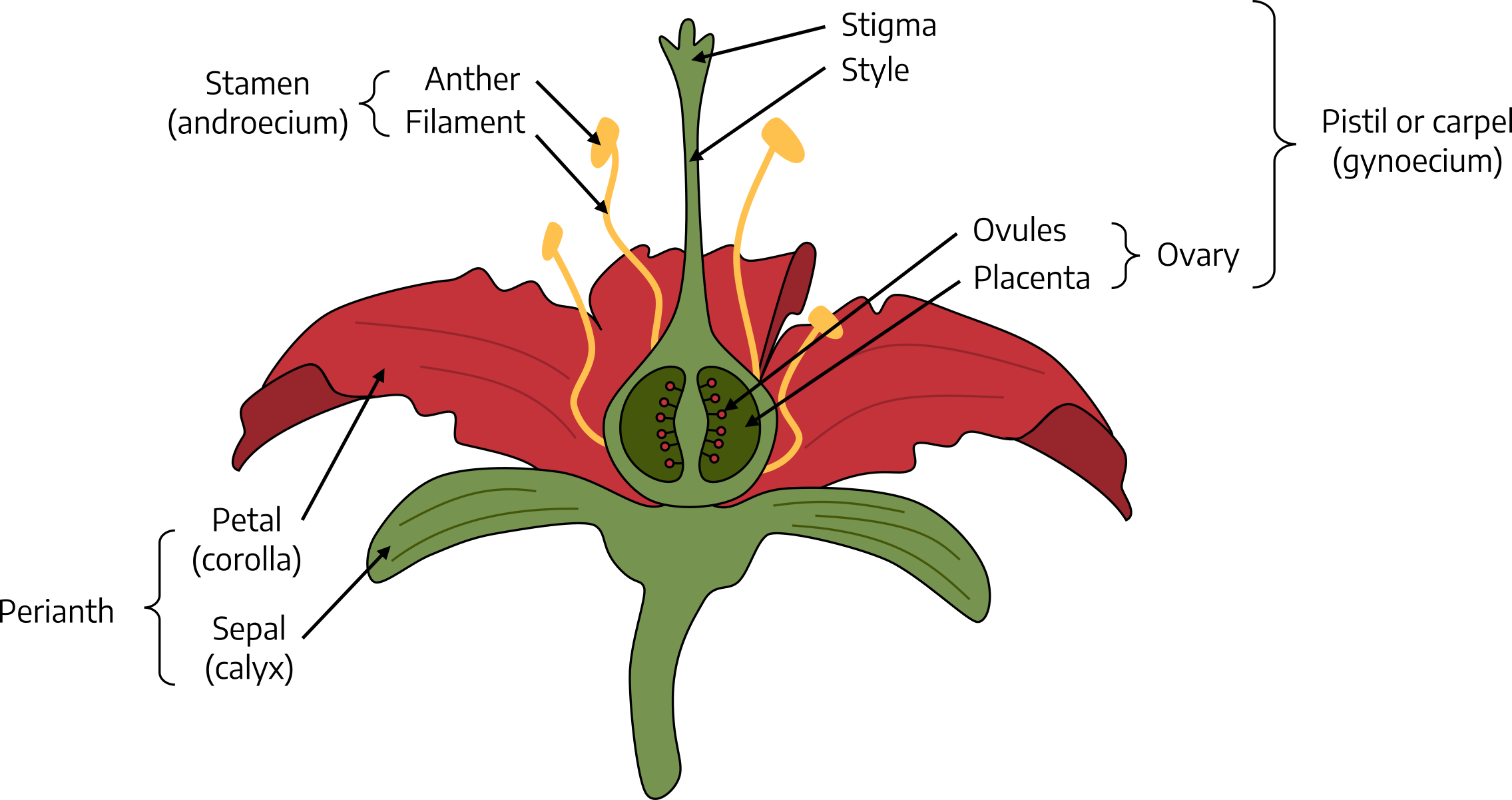
Parts of a flower
As the reproductive part of the plant, the flower contains the male pollen and/or the female ovule plus accessory parts such as petals, sepals, and nectar glands.
Sepals are small, green, leaf-like structures on the base of the flower that protect the flower bud. The sepals collectively are called the calyx.
Petals are highly colored portions of the flower. They may contain perfume as well as nectar glands. The number of petals on a flower is often used in the identification of plant families and genera. The petals collectively are called the corolla. Flowers of dicots typically have sepals and/or petals in multiples of four or five. Monocots typically have these floral parts in multiples of three.
The pistil is the female part of the plant. It is generally shaped like a bowling pin and located in the center of the flower. It consists of the stigma, style, and ovary. The stigma is located at the top and is connected to the ovary by the style. The ovary contains the eggs, which reside in the ovules. After the egg is fertilized, the ovule develops into a seed.
The stamen is the male reproductive organ. It consists of a pollen sac (anther) and a long, supporting filament. This filament holds the anther in position so the pollen it contains may be dispersed by wind or carried to the stigma by insects or birds.
Types of flowers
If a flower has a stamen, pistils, petals, and sepals, it is called a complete flower. If one of these parts is missing, the flower is designated incomplete.
If a flower contains functional stamens and pistils, it is called a perfect flower. (Stamens and pistils are considered the essential parts of a flower.) If either of the essential parts is lacking, the flower is imperfect.
Pistillate (female) flowers are those that possess a functional pistil(s), but lack stamens. Staminate (male) flowers contain stamens, but no pistils.
Because cross fertilization combines different genetic material and produces stronger seed, cross-pollinated plants are usually more successful than self-pollinated plants. Consequently, more plants reproduce by cross pollination than by self pollination.
There are plants that bear only male flowers (staminate plants) or only female flowers (pistillate plants). Species in which the sexes are separated into staminate and pistillate plants are called dioecious. Most hollies trees are dioecious, which means a male and a female plant are necessary to obtain berries. Monoecious plants are those with male and female flower parts on the same plant. Corn plants and pecan trees are examples. Some plants bear only male flowers at the beginning of the growing season, but later develop flowers of both sexes; examples are cucumbers and squash.
How seeds form
Pollination is the transfer of pollen from an anther to a stigma. This may occur by wind or by pollinators. Wind-pollinated flowers lack showy floral parts and nectar since they don’t need to attract a pollinator. Flowers are brightly colored or patterned and contain a fragrance or nectar when they must attract insects, animals, or birds. In the process of searching for nectar, these pollinators will transfer pollen from flower to flower.
The stigma contains a chemical that stimulates activity of pollen from the same type of plant, causing it to grow a long pollen tube down the inside of the style to the ovules inside the ovary. The sperm from the pollen grain moves down the tube, and fertilization typically occurs. Fertilization is the union of the male sperm nucleus (from the pollen grain) and the female egg (in the ovule). If fertilization is successful, the ovule will develop into a seed.
Types of inflorescences
Some plants bear only one flower per stem and are called solitary flowers. Other plants produce an inflorescence, a term that refers to a cluster of flowers and how they are arranged on a floral stem. Most inflorescences may be classified into two groups — racemes and cymes.
In the racemose group, the florets, which are individual flowers in an inflorescence, bloom from the bottom of the stem and progress toward the top. Some examples of racemose inflorescence include spike, raceme, corymb, umbel, and head. A spike is an inflorescence where many stemless florets are attached to an elongated flower stem, or peduncle. A raceme is similar to a spike, except the florets are borne on small stems attached to the peduncle. A corymb is made up of florets with stalks, or pedicels, that are arranged at random along the peduncle in such a way that the florets create a flat, round top. An umbel is similar, except that the pedicels all arise from one point on the peduncle. A head, or composite, inflorescence is made up of numerous stemless florets and is characteristic of daisy inflorescence.
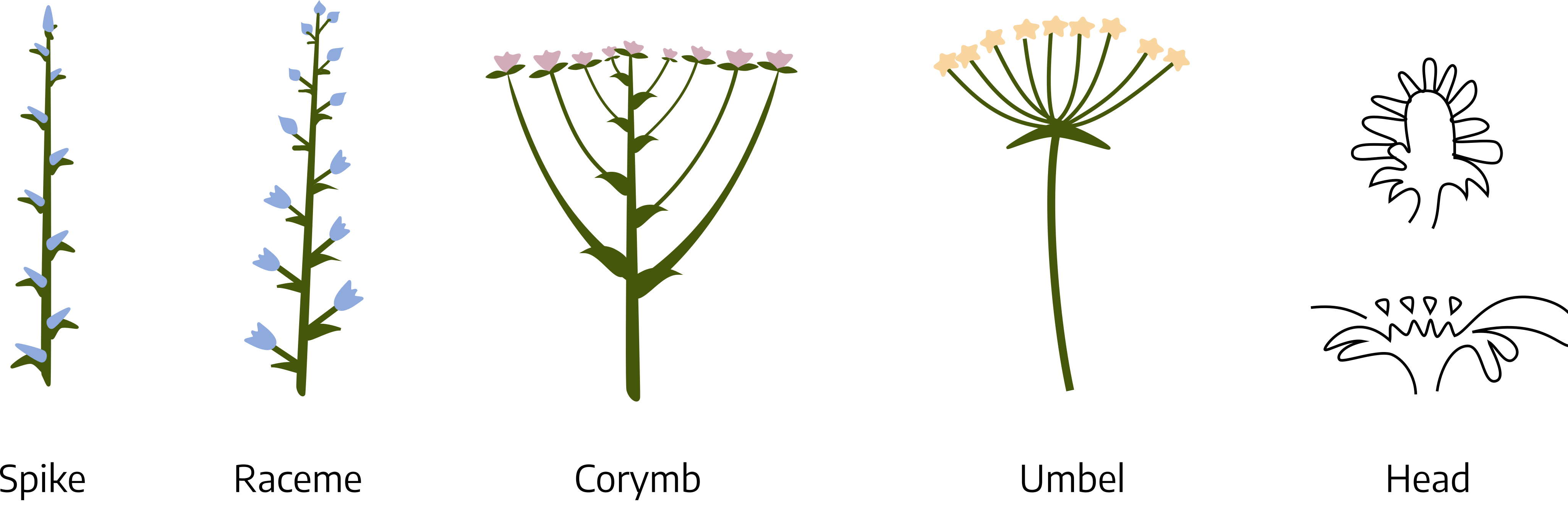
In the cyme group, the top floret opens first and blooms downward along the peduncle. A dichasium cyme has florets opposite each other along the peduncle. A helicoid cyme is one where the lower florets are all on the same side of the peduncle. A scorpioid cyme is one where the florets are alternate to each other along the peduncle.
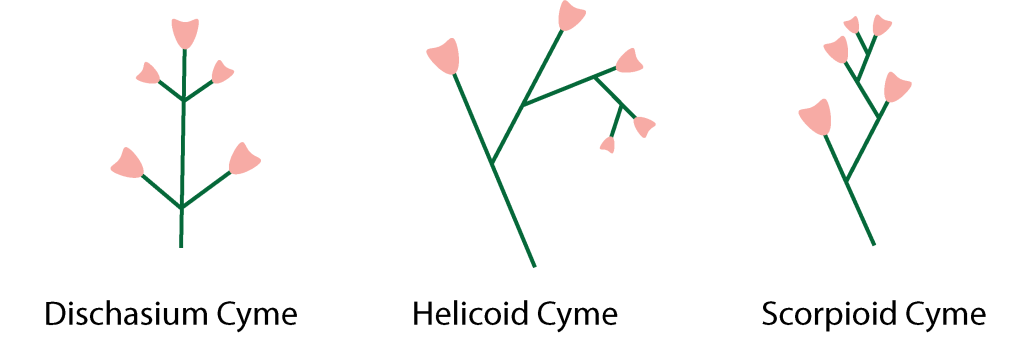
Fruit Anatomy
Parts of fruit
A fruit is a ripened ovary. Fruit consists of the fertilized and mature ovules (called seeds) and the ovary wall, which may be fleshy (as in melons), or dry and hard (as in a pecan nut). The only parts of the fruit that are genetically representative of both the male and female flowers are the seeds (mature ovules). The rest of the fruit arises from the maternal plant, and is therefore genetically identical to that parent. Many fruits have additional maternal tissues develop along with the ovary; for example, the core of an apple is the ovary containing the seeds, while the part consumed develops from the receptacle that supported the ovary in the flower.
Types of fruit
Fruits can be classified as simple fruits, aggregate fruits, or multiple fruits. Simple fruits develop from a single ovary. There are several types of simple fruits:
- Berry: Fleshy pericarp (ovary) surrounding the seeds (e.g., tomatoes, grapes, eggplant, blueberries)
- Drupe: Hard, stony endocarp (where seed is located) surrounded by fleshy mesocarp (middle section of ovary) like a peach
- Pepo: A modified berry with a hard rind (e.g., cucumbers, pumpkin, watermelon)
- Legume: A simple fruit that splits along 2 seams (e.g., bean, peanut)
- Achene: Small, thin-walled (papery seed) dry fruit that does not spit open at maturity (e.g., dandelion, strawberry seeds)
- Pome: A type of accessory fruit where the edible part is a swollen receptacle and the center consists of fused carpels
- Samsara: A winged achene
- Nut: A seed surrounded by a thick, hard ovary wall (pericarp) (e.g. acorns and chestnuts are true nuts)
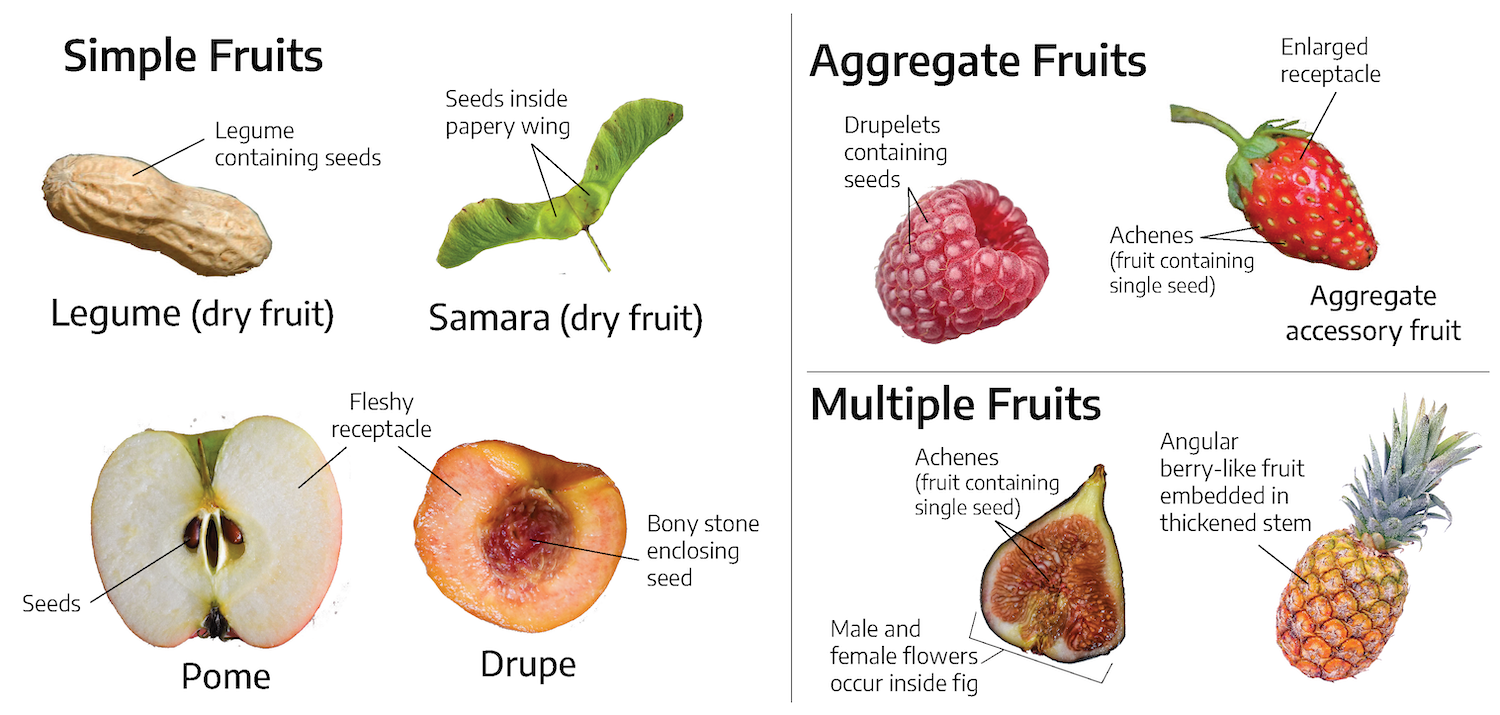
Aggregate fruits, such as raspberries, come from a single flower with many ovaries. The flower appears as a simple flower with one corolla, one calyx, and one stem, but with many pistils or ovaries. The ovaries are fertilized separately and independently. If ovules are not pollinated successfully, the fruit will be misshapen and imperfect. Strawberry and blackberry are also aggregate fruits with the addition of an edible, enlarged receptacle. For this reason, they are sometimes termed aggregate-accessory fruits.
Multiple fruits are derived from a tight cluster of separate, independent flowers borne on a single structure. Each flower has its own calyx and corolla. Examples of multiple fruits are pineapple, fig, and the beet seed. Multiple fruits are not common in Virginia.
Seed Anatomy
The seed, or matured ovule, is made up of three parts. The embryo is a miniature plant in an arrested state of development. Most seeds contain a built-in food supply called the endosperm which can be made up of proteins, carbohydrates, or fats. (Orchid is an exception in producing no endosperm, while in some other mature seeds, the endosperm has been absorbed and stored within the embryo.) The third part is the hard outer covering, called a seed coat, which protects the seed from disease and insects and prevents water from entering the seed (this would initiate the germination process before the proper time).
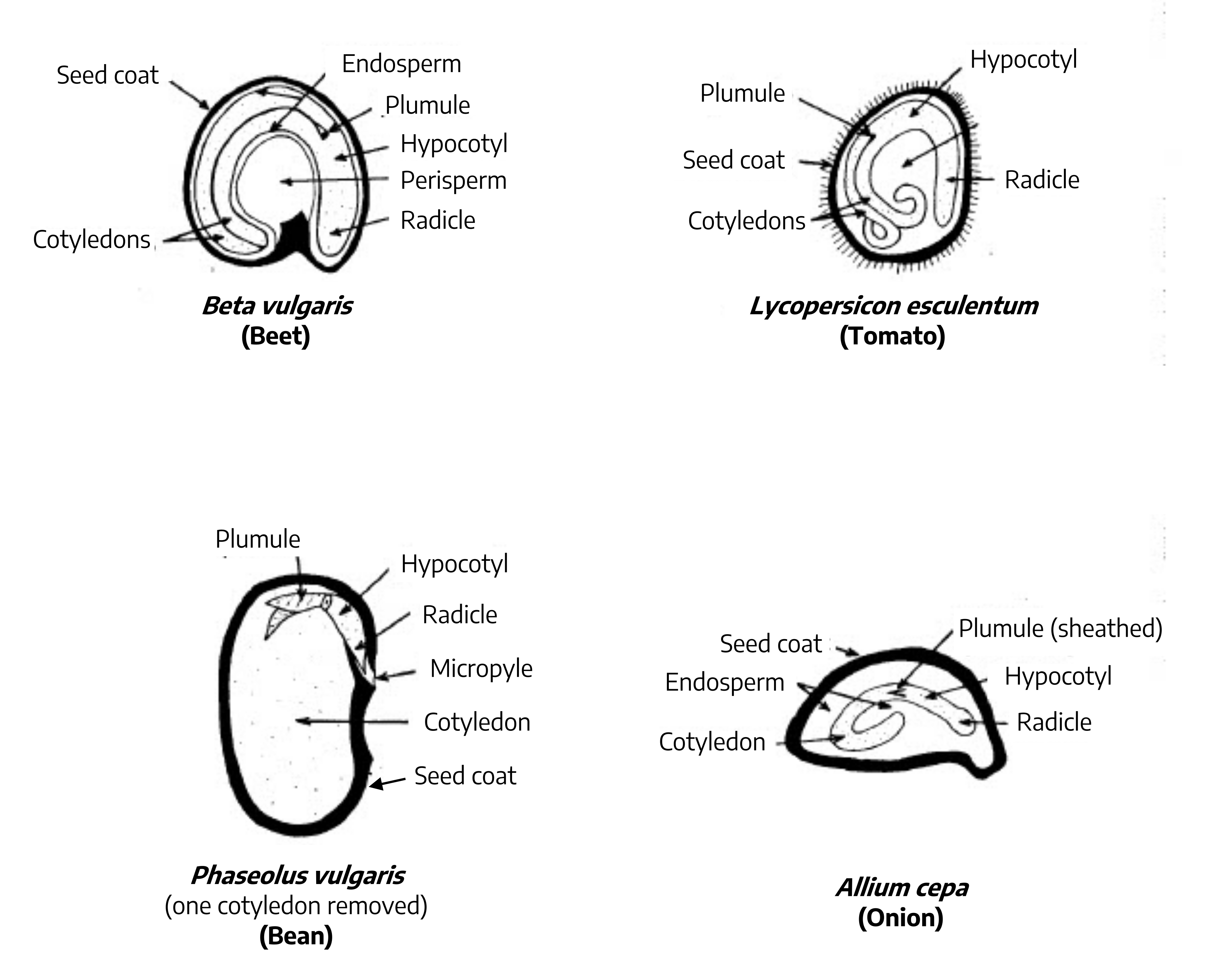
Seedlings
Germination is the resumption of active embryo growth. Prior to any visual signs of growth, the seed must absorb water through the seed coat. In addition, the seed must be in the proper environmental conditions; that is, exposed to oxygen, favorable temperatures, and for some, correct light. The radicle is the first part of the seedling to emerge from the seed. It will develop into the primary root from which root hairs and lateral roots develop. The portion of the seedling between the radicle and the first leaf-like structure is called the hypocotyl. Above the hypocotyl is the plumule, which is the embryonic shoot. The seed leaves, or cotyledons, encase the embryo and are usually different in shape from the leaves that the mature plant will produce. Plants producing one cotyledon fall into the group of monocotyledons or monocots. Plants producing two seed leaves are called dicotyledons or dicots. A micropyle a small pore located in the seedcoat that allows water absorption and gas exchange.
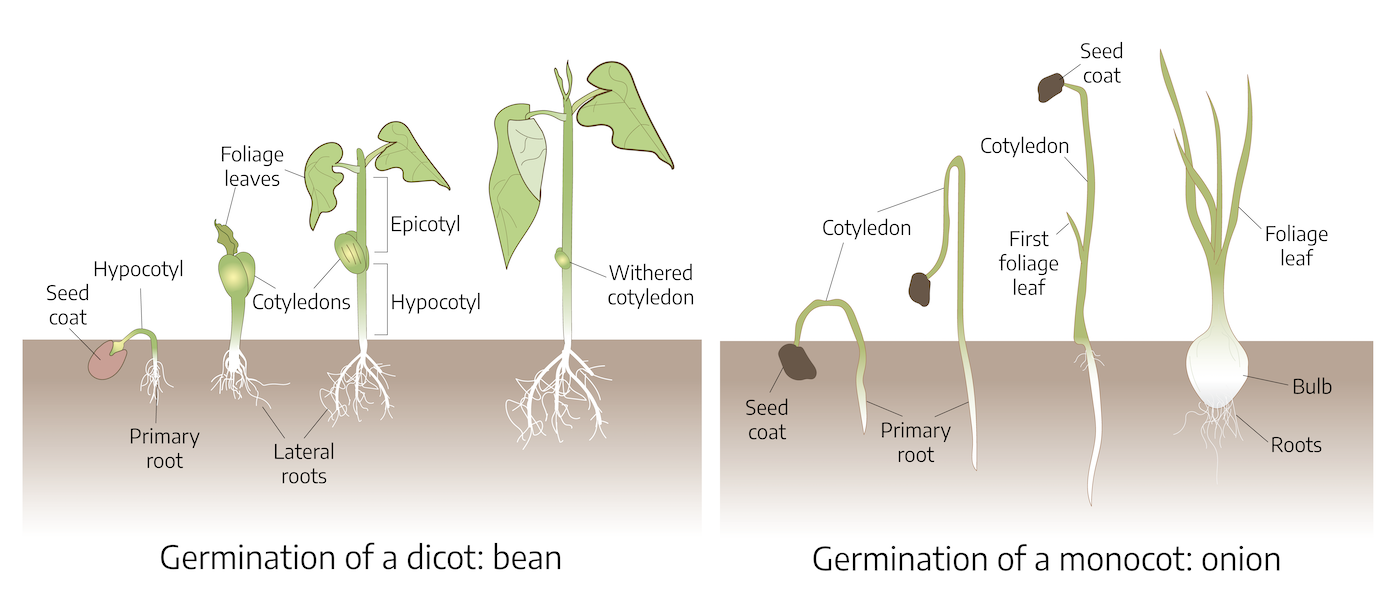
Green Spring Gardens “Planting Seeds of Hope” A Master Gardener approach to Therapeutic Gardening
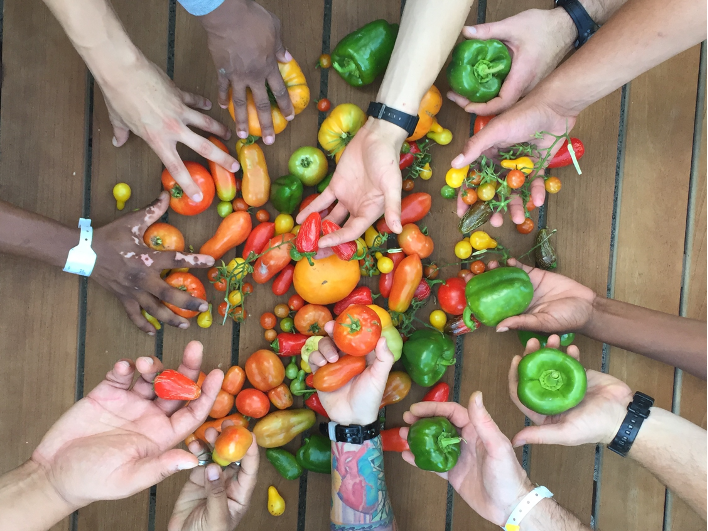
By Kathleen Wellington, Extension Master Gardener, Green Spring Gardens
Since 2016, Green Spring Extension Master Gardeners in Fairfax County have implemented and sustained on-going therapeutic gardening projects. Green Spring Extension Master Gardeners (GSEMG’s) provide education and therapeutic focused hands-on gardening experiences for individuals with mental health and substance abuse challenges at the Fairfax County Wellness Circle Crisis Stabilization Program and at the USO Resiliency Garden at Fort Belvoir.
During the initial phases of implementing these projects, the GSEMG’s met with unanticipated challenges that jeopardized the success of the projects. Staff uninterested in gardening, lack of management support, along with a lack of understanding of the benefits of gardening for individuals with special needs were just some of the challenges encountered.
Out of these challenges originated Green Spring’s “Planting Seeds of Hope” initiative: a replicable framework to implement successful gardening for individuals with special needs.
Here are some examples of things that have made our horticulture therapy projects successful:
- Master Gardener and program staff training – including modules pertaining to definition and theoretical information about horticulture therapy, benefits of gardening, and practical applications to special populations for GSEMG’s. Program staff training includes an understanding of the mission of the Master Gardener program and education on therapeutic gardening and its relevance to the program objectives.
- Development of project goals – developed with program staff consistent with the needs of the clients, along with a plan to implement and measure progress.
- Therapeutic focused education and hands-on gardening that is not “show or tell” but “do with” education adapted to the comprehension level of clients.
- Development of self-sustaining gardening manuals for the program, including “how to” garden education that connects the task with the therapeutic/wellness benefits.
- Coordination with staff recognizing that staff are experts on their clients but GSEMG’s can help staff work more successfully with clients through therapeutic gardening techniques (sensory stimulation, development of skill sets, metaphors, etc.).
Sustainable funding/community partnerships including awareness of the difference in fundraising guidelines between nonprofits and government entities. Collaboration with program management to understand the best opportunities for funding such as inclusion in program budget, fund raising, partnerships with community organizations and the value of media recognition.
Physiology: Plant Growth and Development
The five major plant functions that are the basics for plant growth and development are photosynthesis, respiration, transpiration, absorption, and translocation.
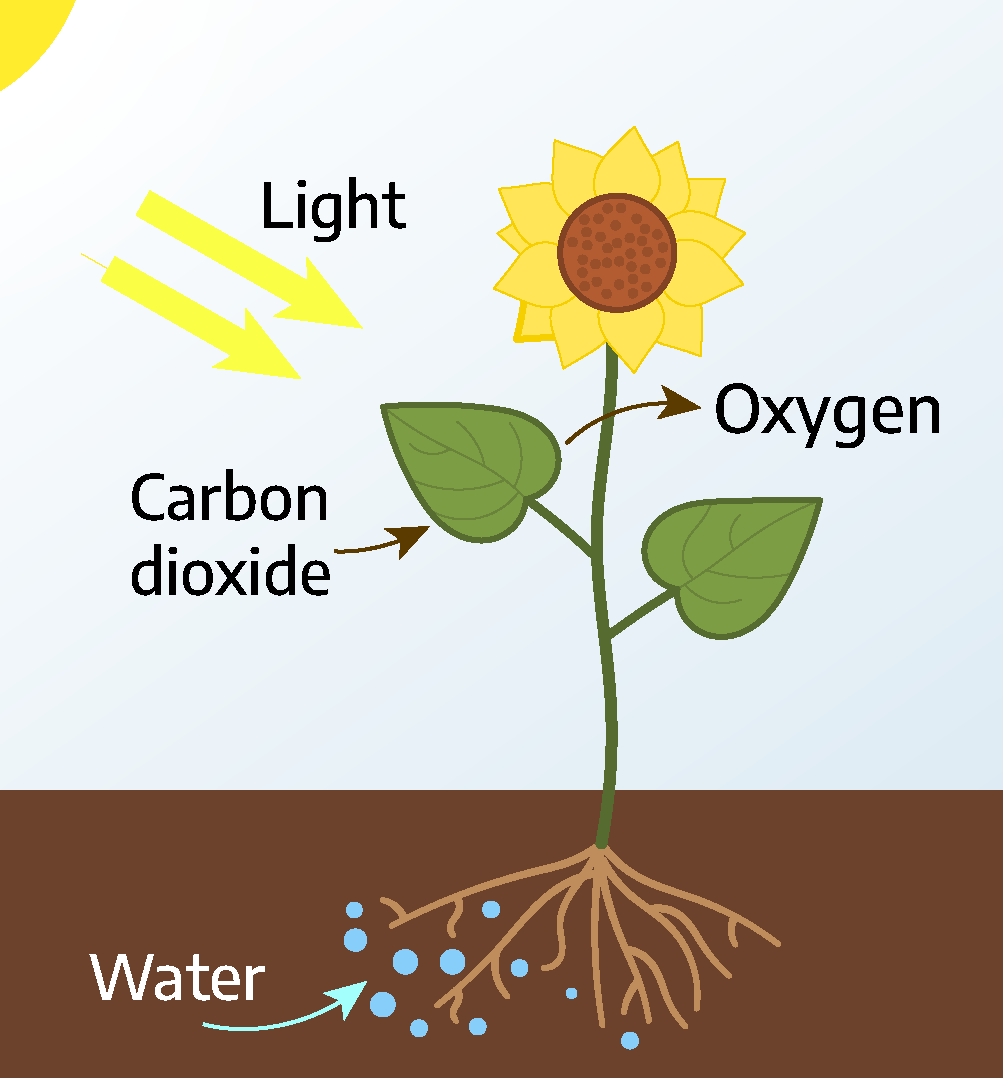
Photosynthesis
One of the major differences between plants and animals is the ability of plants to internally manufacture their own food (called autotrophy). To produce food for itself, a plant requires energy from sunlight, carbon dioxide from the air, and water from the soil. If any of these ingredients is lacking, photosynthesis, or food production, will stop. If any factor is removed for a long period of time, the plant will die. Photosynthesis literally means “to put together with light.”
6 CO2 + 6 H20 + sunlight [latex]\longrightarrow[/latex] C6H12O6 + 6 O2
Plants first store the energy from light in simple sugars, such as glucose (C6H12O6). Some of these sugars are converted back to water and carbon dioxide, releasing the stored energy through the process called respiration. This energy released from respiration is required for all living processes and growth. Simple sugars are also converted to other sugars and starches (carbohydrates) which may be transported to the stems and roots for use or storage, or may be used as building blocks for more complex structures (e.g., oils, pigments, proteins, cell walls).
Any green plant tissue is capable of photosynthesis. Chloroplasts in these cells contain the green pigment chlorophyll which traps the light energy. However, leaves are generally the site of most food production due to their special structure. The internal tissue (mesophyll) contains cells with abundant chloroplasts in an arrangement that allows easy movement of water and air. The protective upper and lower epidermis (skin) layers of the leaf include many stomata that regulate movement of the gases involved in photosynthesis into and out of the leaf.
Photosynthesis is dependent on the availability of light. Generally speaking, as sunlight increases in intensity, photosynthesis increases. This results in greater food production. Many garden crops, such as tomatoes, respond best to maximum sunlight. Tomato production is cut drastically as light intensities drop. Only two or three varieties of “greenhouse” tomatoes will produce any fruit when sunlight is minimal in late fall and early spring.
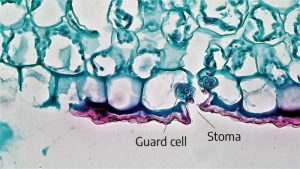
Water (H2O) plays an important role in photosynthesis in several ways. First, it maintains a plant’s turgor, the firmness or fullness of plant tissue. Turgor pressure in a cell can be compared to air in an inflated balloon. Water pressure or turgor is needed in plant cells to maintain shape and ensure cell growth. Second, water is split into hydrogen and oxygen by the energy of the sun that has been absorbed by the chlorophyll in the plant leaves. The oxygen (O2) is released into the atmosphere, and the hydrogen is used in manufacturing carbohydrates. Third, water dissolves minerals from the soil and transports them up from the roots and throughout the plant where they serve as raw materials in the growth of new plant tissues. The soil surrounding a plant should be moist, not too wet or too dry. Water is pulled through the plant by evaporation of water through the leaves (transpiration).
Photosynthesis also requires carbon dioxide (CO2) which enters the plant through the stomata. Carbon and oxygen are used in the manufacturing of carbohydrates. Carbon dioxide in the air is plentiful enough so that it is not a limiting factor in plant growth. However, since carbon dioxide is consumed in making sugars and is not released by plants at an equal rate, a tightly closed greenhouse in midwinter may not let in enough outside air to maintain an adequate carbon dioxide level. Under these conditions, improved crops of roses, carnations, tomatoes, and certain other crops can be produced if the carbon dioxide level is raised with CO2 generators or, in small greenhouses, with dry ice.
Although not a direct component in photosynthesis, temperature is an important factor. Photosynthesis occurs at its highest rate in the temperature range 65 to 85°F (18 to 27°C) and decreases when temperatures are above or below this range.
Respiration
Carbohydrates made during photosynthesis are of value to the plant when they are converted into energy. This energy is used in the process of building new tissues (plant growth). The chemical process by which sugars and starches produced by photosynthesis are converted into energy is called respiration. It is similar to the burning of wood or coal to produce heat (energy). This process in cells is shown most simply as:
C6H12O6 + 6 O2[latex]\longrightarrow[/latex] 6 CO2 + 6 H2O + Energy released
This equation is precisely the opposite of that used to illustrate photosynthesis, although more is involved than just reversing the reaction. However, it is appropriate to relate photosynthesis to a building process, while respiration is a breaking-down process.
Table 1-1: Photosynthesis vs respiration
Photosynthesis | Respiration |
---|---|
Produces food | Uses food for plant energy |
Stores energy | Releases energy |
Occurs in cells containing chloroplasts | Occurs in all cells |
Releases oxygen | Uses oxygen |
Uses water | Produces water |
Uses carbon dioxide | Produces carbon dioxide |
Occurs in sunlight | Occurs in darkness and light |
By now, it should be clear that respiration is the reverse of photosynthesis. Unlike photosynthesis, respiration occurs at night as well as during the day. Respiration occurs in all life forms and in all cells. The release of accumulated carbon dioxide and the uptake of oxygen occurs at the cell level. In animals, blood carries both carbon dioxide and oxygen to and from the atmosphere by means of the lungs or gills. In plants, there is simple diffusion into the open spaces around the cells, and exchange occurs through the stomata on leaves and stems, or through root hairs.
Transpiration
Transpiration is the process by which a plant loses water, primarily from leaf stomata. Transpiration is a necessary process that involves the use of about 90% of the water that enters the plant through the roots. The other 10% of the water is used in chemical reactions and in plant tissues. Transpiration is involved in the movement of water, minerals, and at times, stored sugars from the roots to other parts of the plant. This occurs in the xylem.
Transpiration and turgor pressure: The amount of water lost from the plant depends on several environmental factors such as temperature, humidity, and wind or air movement. An increase in temperature or air movement decreases humidity outside the leaf and increases the rate of transpiration. This presents a continuing danger to plants as the rate of water loss may not be matched by the rate of water absorption from dry soil into the roots. A water deficit in the plant may only lead to temporary wilting (loss of turgor pressure) from which the plant may rapidly recover when the transpiration rate decreases later in the day or stops overnight. The guard cells respond to the loss of turgor by shrinking and closing the stomata. While this significantly reduces further damaging water loss, it also impedes carbon dioxide entry for photosynthesis. Repeated temporary wilting can lead to stunted plants due to reduction of the food supply and other metabolic changes, especially in cell division and enlargement. A plant maintains adequate turgor pressure when the amount of water loss due to transpiration is equal to the amount of water absorbed into the plant.
Absorption
Absorption is the process by which substances, particularly water and minerals, are moved into the plant. This occurs mainly through the roots in the tip region where root hairs are present, but it may also occur through leaf surfaces. Water absorption into roots may be a passive process due to a “pulling” action, drawing water through the xylem tubes to replace water lost from the leaves through transpiration. Other water absorption is an active process linked to active absorption of mineral nutrients. This is discussed later in the section on plant nutrition.
Translocation
Translocation is the movement of sugars, amino acids, and other plant chemicals from the leaves to other parts of the plant through the phloem. This translocation is often an active process requiring respiration energy as the substances are moved upward and downward in the plants to growing areas or storage.
Environmental Factors Affecting Plant Growth
Plant growth and distribution are limited by the environment. If any one environmental factor is less than ideal, it will become a limiting factor in plant growth. Limiting factors are also responsible for the geography of plant distribution. For example, only plants adapted to limited amounts of water can live in deserts. Most plant problems are caused by environmental stress, either directly or indirectly. Therefore, it is important to understand the environmental aspects that affect plant growth. These factors are light, temperature, water, humidity, and nutrition. For more about how these factors impact plants, see Chapter 5: “Abiotic Stress.”
Light
Light has three principal characteristics that affect plant growth: quantity, quality, and duration.
Light quantity refers to the intensity or concentration of sunlight and varies with the season of the year. The maximum is present in the summer and the minimum in winter. The more sunlight a plant receives (up to a point), the better capacity it has to produce plant food through photosynthesis. As the sunlight quantity decreases, the photosynthetic process decreases. Light quantity can be decreased in a garden or greenhouse by using cheesecloth shading above the plants. It can be increased by surrounding plants with white or reflective material, or supplemental lights.
Light quality refers to the color or wavelength reaching the plant surface. Sunlight can be broken up by a prism into respective colors of red, orange, yellow, green, blue, indigo, and violet. On a rainy day, raindrops act as tiny prisms and break the sunlight into these colors, producing a rainbow. Red and blue light have the greatest effect on plant growth. Green light is least effective to plants as they reflect green light and absorb none. It is this reflected light that makes them appear green to us. Blue light is primarily responsible for vegetative growth or leaf growth. Red light, when combined with blue light, encourages flowering in plants. Fluorescent, or cool-white, light is high in the blue range of light quality and is used to encourage leafy growth. Such light would be excellent for starting seedlings. Incandescent light is high in the red or orange range, but generally produces too much heat to be a valuable light source. Fluorescent “grow” lights have a mixture of red and blue colors that attempts to imitate sunlight as closely as possible, but they are costly and generally not of any greater value than regular fluorescent lights.
Light duration, or photoperiod, refers to the amount of time that a plant is exposed to sunlight. When the concept of photoperiod was first recognized, it was thought that the length of periods of light triggered flowering. The various categories of response were named according to the light length (i.e., short-day and long-day). It was then discovered that it is not the length of the light period, but the length of uninterrupted dark periods that is critical to floral development. The ability of many plants to flower is controlled by photoperiod. Plants can be classified into three categories depending upon their flowering response to the duration of darkness. These are short-day, long-day, or day-neutral plants.
Short-day plants form their flowers only when the day length is less than about 12 hours in duration. Short-day plants include many spring- and fall-flowering plants, such as chrysanthemum and poinsettia. Long-day plants form flowers only when day lengths exceed 12 hours (short nights). They include almost all of the summer-flowering plants, such as rudbeckia and California poppy, as well as many vegetables, including beet, radish, lettuce, spinach, and potato. Day-neutral plants form flowers regardless of day length. Some plants do not really fit into any category, but may be responsive to combinations of day lengths. The petunia will flower regardless of day length, but flowers earlier and more profusely under long daylight. Since chrysanthemums flower under the short-day conditions of spring or fall, the method for manipulating the plant into experiencing short days is very simple. If long days are predominant, a shade cloth is drawn over the chrysanthemum for 12 hours daily to block out light until flower buds are initiated. To bring a long-day plant into flower when sunlight is not present longer than 12 hours, artificial light is added until flower buds are initiated.
Temperature
Temperature affects the productivity and growth of a plant, depending on whether the plant variety is a warm- or cool-season crop. If temperatures are high and day length is long, a cool-season crop such as spinach will bolt (flower prematurely) rather than produce the desired flower. Temperatures that are too low for a warm-season crop such as tomato will prevent fruit set. Adverse temperatures also cause stunted growth and poor quality. For example, the bitterness in lettuce is caused by high temperatures.
Sometimes temperatures are used in connection with day length to manipulate the flowering of plants. Chrysanthemums will flower for a longer period of time if daytime temperatures are around 59°F (15°C). The Christmas cactus forms flowers as a result of short days and low temperatures. Daffodils are forced to flower by putting the bulbs in cold storage in October at 35 to 40°F (2 to 4°C). The cold temperatures allow the bulb to break dormancy. The bulbs are transferred to the greenhouse in midwinter where growth begins. The flowers are then ready for cutting in three to four weeks.
Thermoperiod refers to the daily range of temperatures a plant is exposed to. Plants produce maximum growth when exposed to a day temperature that is about 10 to 15° higher than the night temperature. This allows the plant to photosynthesize (build up) and respire (break down) during an optimum daytime temperature and to curtail the rate of respiration during a cooler night. High temperatures cause increased respiration, sometimes above the rate of photosynthesis. This means that the products of photosynthesis are being used more rapidly than they are being produced. This causes plant growth to slow down or even stop. For growth to occur, photosynthesis must be greater than respiration.
Low temperatures can result in poor growth. Photosynthesis is slowed down at low temperatures. Since photosynthesis is slowed, growth is slowed, and this results in lower yields. Not all plants grow best in the same temperature range. For example, snapdragons grow best when night temperatures are 55°F (12°C); the poinsettia prefers 62°F (17°C). Florist cyclamen does well under very cool conditions, while many bedding plants prefer a higher temperature. Recently, it has been found that roses can tolerate much lower night temperatures than previously believed. This has meant a conservation in energy for greenhouse growers.
However, in some cases, a certain number of days of low temperatures are needed by plants to grow properly. This is true of crops growing in cold regions of the country. Peaches are a prime example; most varieties require 700 to 1000 hours between 45°F (7°C) and 32°F (0°C) before they break their rest period and begin growth. Lilies need 6 weeks at 33°F (1°C) before blooming.
Plants can be classified as either hardy or nonhardy (tender), depending on their ability to withstand cold temperatures. Winter injury can occur to nonhardy plants if temperatures are too low or if unseasonably low temperatures occur early in the fall or late in the spring. Winter injury may also occur because of desiccation (drying out) — plants need water during the winter. When the soil is frozen, the movement of water into the plant is severely restricted. On a windy winter day, broadleaved evergreens can become water-deficient in a few minutes; the leaves or needles then turn brown. Wide variations in winter temperatures can cause premature bud break in some plants and consequent bud-freezing damage. Late spring frosts can ruin entire peach crops. If temperatures drop too low during the winter, entire trees of some species are killed by the freezing and splitting of plant cells and tissue.
Water
Water is a primary component of photosynthesis. It maintains the turgor pressure or firmness of tissue and transports nutrients throughout the plant. In maintaining turgor pressure, water is the major constituent of the protoplasm (living material) of a cell. By means of turgor pressure and other changes in the cell, water regulates the opening and closing of the stoma, thus regulating transpiration. Water also provides the pressure to move a root through the soil. Among water’s most critical roles is that of the solvent for minerals moving into the plant and for carbohydrates moving to their site of use or storage.
Relative humidity is the ratio of water vapor in the air to the amount of water the air could hold at a given temperature and pressure, expressed as a percent.
For example, if a kilogram of air at 75°F could hold 4 grams of water vapor and there are only 3 grams of water in the air, then the relative humidity (RH) is:
Expressed as a percent = 75%
Warm air can hold more water vapor than cold air; therefore, if the amount of water in the air stays the same and the temperature increases, the relative humidity decreases.
Water vapor will move from an area of high RH to one of low RH. The greater the difference in humidity, the faster water will move.
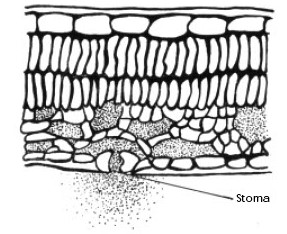
The relative humidity in the air space between the cells within the leaf approaches 100%; therefore, when the stomate is open, water vapor rushes out. As the vapor moves out, a cloud of high humidity is formed around the stomate. This cloud of humidity helps slow down transpiration and cool the leaf. If air movement blows the humid cloud away, transpiration will increase.
Nutrition
Many people confuse plant nutrition with plant fertilization. Plant nutrition refers to the needs and uses of the basic chemical elements in the plant. Fertilization is the term used when these materials are supplied to the environment around the plant. A lot must happen before a chemical element supplied in a fertilizer can be taken up and used by the plant.
Plants need 16 elements for normal growth. Carbon, hydrogen, and oxygen are found in air and water. Nitrogen, potassium, magnesium, calcium, phosphorous, and sulfur are found in the soil. These six elements are used in relatively large amounts by the plant and are called macronutrients (nitrogen, potassium, and phosphorous are the primary macronutrients and magnesium, sulfur and calcium are the secondary macronutrients). There are seven other elements called micronutrients (or trace elements) and are used in much smaller amounts. Micronutrients are found in the soil: iron, zinc, molybdenum, manganese, boron, copper, and chlorine. All 16 elements, both macronutrients and micronutrients, are essential for plant growth.
Most of the nutrients that a plant needs are dissolved in water and then absorbed by the roots. Many nutrient combinations in fertilizers dissolve easily, and those nutrients can be readily absorbed. Sometimes two dissolved nutrients will combine into a product that has very low solubility. Availability of both nutrients to the plant is then severely reduced. This can occur with calcium and phosphorus and the micronutrients. Nutrient solubility is also affected by soil pH. High (alkaline) pH levels drastically reduce the solubility and availability of micronutrients (a factor in iron deficiency in azaleas), while low (very acidic) pH levels make some micronutrients (and non-nutrient minerals such as aluminum) so highly available as to injure the plant. Another consideration is the nutrient balance in the soil. For example, calcium and magnesium are absorbed similarly, but magnesium is absorbed more readily. The root does not select the nutrient to be absorbed; if both are present at the absorption site, the magnesium will be absorbed. This is why a soil test may indicate that, while there is sufficient calcium in the soil, a plant can suffer calcium deficiency because of an excess of magnesium competing for absorption.
The process whereby nutrients are absorbed varies. Water and nutrients can move between the outer cells of the root, but eventually they must cross a membrane to enter a cell. Water and some nutrients can do this easily (a passive process) while other nutrients are too large for the ‘holes’ in the membrane, and energy is needed to move these nutrients into the cell (an active process). Absorption is generally a combination of these processes: certain nutrients are actively absorbed, others enter passively to maintain a chemical balance.
Anything that lowers or prevents the production of sugars in the leaves can lower nutrient absorption. If the plant is under stress due to low light or extremes in temperature, nutrient deficiency problems may develop. The stage of growth or rate of growth may also affect the amount of nutrients absorbed. Many plants go into a rest period, or dormancy, during part of the year. During this dormancy, few nutrients are absorbed. Plants may also absorb different nutrients just as flower buds begin to develop.
Foliar absorption: A special case
Under normal growing conditions, plants absorb most nutrients except carbon, hydrogen, and oxygen from the soil. However, some nutrients can also be absorbed by the leaves if they are sprayed with a dilute solution. The factors that affect absorption by the cell are still important because the nutrient must enter the cell to be used by the plant. Care must be taken that the concentration of the nutrient is not too high or the leaf will be injured. The nutrient must get around or through the waxy leaf cuticle before it can enter the cell.
Taxonomy: Biological Classification
Taxonomy is the science of biological classification of plants and animals. The purpose of taxonomy is to develop a convenient and precise method of classifying human knowledge. This method thus preserves knowledge and makes it accessible. In this chapter, we will learn how plants are classified, then learn how to go about identifying a plant by the use of a leaf key.
Classification of Landscape Plants
There are many ways to classify or categorize the thousands of plants used in the landscape. The validity of any system depends on consistency, clarity, and utility. For example, the classification of plants based on use, such as ornamentals in contrast to edibles, seems logical until you consider some species which are both ornamental and edible. Other ways of classifying plants, such as through the relationships of their growth habits, are also descriptive though frequently not a basis for easy differentiation. For example, differences between some trees and shrubs or between evergreen, semi-evergreen, and deciduous trees and shrubs may depend on geographical location. Other divisions include length of life (annual, biennial, or perennial) and type of stem tissue (woody or herbaceous).
Among woody plants, a major distinction is made between those that lose their leaves during part of the year (deciduous) and those with leaves that persist during the entire year (evergreen). There are also those that hold their leaves late into the winter when others are leafless, but eventually lose their leaves or turn brown (semi-evergreen). In fact, even evergreen plants ultimately lose their leaves, though it is usually after new leaves have been formed. Some evergreens hold their leaves only one year, but most hold them two or more years.
In addition to the very general horticultural classifications outlined previously, plants are further differentiated for landscape purposes by size, growth habit, usage, and adaptability to specific environmental conditions. See Chapter 16: “Landscape Design” for more information.
Finally, landscape plants are classified according to tolerance of various environmental conditions or on the basis of their requirement of certain conditions. This is particularly true of plants with growth that is unique to a specific habitat, such as bog plants and indoor or outdoor plants. Major categories related to environment are tropical, subtropical, and temperate. Within each of these categories and locations, plants are classified either as hardy or tender, depending on whether or not the plant will survive the winter of a particular region. These, along with the scientific classification, ought to provide you with sufficient background to build upon as you become increasingly experienced.
Scientific Classification
Various methods of classifying plants have been used throughout history, including some of the approaches already mentioned; however, it was the effort of Carolus Linnaeus (1707-1778) that revolutionized plant classification and gave form to the present scientific system. This system uses structural (morphological) similarities and differences, particularly in the reproductive organs, as a basis for classification. These plant parts are the least likely to be influenced by environmental conditions and, therefore, provide stable distinguishing features.
Plant classification begins by dividing the plant kingdom into major divisions, separated on an evolutionary basis. The division that will be of importance to you is the most advanced division containing the so-called higher plants. This division, known as Tracheophyta, are plants with roots, stems, leaves, and vascular systems. Further divisions can be illustrated by using as an example a cultivar of beautyberry called ‘Early Amethyst’: Callicarpa dichotoma ‘Early Amethyst.’
Callicarpa dichotoma ‘Early Amethyst’ is described by each of the following categories. The precision of the description increases as the list descends.
Kingdom: Plant
– Division: Tracheophyta
— Class: Magnoliopsida
—- Order: Lamiales
—– Family: Lamiaceae
—— Genus: Callicarpa
——- Species: C. dichotoma
——– Cultivar: ‘Early Amethyst’
The family has very specific distinguishing characteristics that can be used for identification purposes. This is particularly true of reproductive (flower) parts, but frequently holds true for non-reproductive structural features such as leaf and bud arrangements. Family names end in aceae. For example, apples, cherries, and peaches are in the Rosaceae family (rose family). Historically, plants have been grouped into families based on flower and fruit characteristics; currently, DNA sequencing is being used to clarify the true identity of plants and their relationships to other species/genera. While the family concept is not generally used in the nursery and landscape industries, knowledge of the family can prove to be helpful. For example, species in the Rosacea family may be prone to pest problems, and species in the Ericaceae (heath) family (e.g., azaleas and blueberries) generally require well drained acid soils.
Below family, plants are grouped in a particular genus and these plants are very similar morphologically. Sometimes members of the same genus but different species can cross pollinate among themselves.
Below genus the basic unit of this taxonomy system is the species. Distinct and repeated variation within a species is often observed. This results in the naming of a subspecies or variety or cultivar (cultivated variety). A hybrid is a genetic cross of two different plants, usually from two different varieties of the same species. The following discussion will help you understand the binomial system of nomenclature. Note that the species and variety names always include the genus name.
Common Plant Names
All plants bear at least one common name, a vernacular name that is commonly attached to a plant. Examples of common names are red maple, American beech, white pine, and redbud. The issues of using these familiar names are that 1) a plant often has more than one common name, 2) names can vary from region to region, and 3) names vary from one language to another. Furthermore, two completely unrelated tree species can share the same or similar common name. For example, the common name of a conifer commonly seen throughout Virginia is eastern red cedar (Juniperus virginiana). Eastern red cedar is a juniper and not a type of cedar; it is not even in the same plant family as a true cedar (genus = Cedrus). Thus, referring to common names in horticultural plant commerce is a very unreliable way to describe a plant.
Plants also have a scientific name which is essential to correctly identify, propagate, sell, purchase, use, assess, and diagnose plants in many horticultural applications. Most wholesale plant catalogs list plants by scientific name, and anyone in the horticultural realm needs to know plants by their scientific name. Scientific names are “relatively” stable; botanists occasionally reclassify plants resulting in family and species names being changed. In general, common names are written in lower case with the exception of proper names (e.g., American beech).
Many garden centers and virtually all wholesale plant vendors list their plants by the scientific name, so knowing both names is important. Knowing plant names also helps you understand the relationships (plant growth relative to light, water, soil, and climate aspects) between plants when observing plants in natural or man-made landscapes. The use of a plant’s scientific name is the best and most reliable way to describe a plant.
Scientific Names
Genus and Specific Epithet = Species
All known living things are given a two-word Latin scientific name, a generic name followed by a specific name. Latin was chosen because it is a dead language (not commonly spoken and no longer evolving). This two-word name (binomial) is descriptive of only one plant (i.e., species) and is composed of a genus and a specific epithet (species). For example, humans are Homo sapiens; Homo is the genus (a generic name) and sapiens is the specific epithet (a specific name). Collectively, the genus followed by the specific epithet is a species.
The scientific name is very important to know in most situations since there is no ambiguity on the interpretation of the name. For example, the common name of Acer rubrum is red maple. However, red maple is also called scarlet maple and swamp maple. Adding to the confusion is that there are a few maples that can have red foliage. However, if the Acer rubrum name is used, then one knows exactly which species is being referred to.
A genus is a group of somewhat closely related individuals (a group name) comprising one or more species. For example, the genus name for all maples is Acer, and the genus name for all oaks is Quercus. A genus name is always a noun and a single species name is either an adjective or another supporting noun. The specific epithet relates to such things as plant attributes, the place it was originally found, or the discoverer. The specific epithet is the second word of the Latin binomial that usually functions as an adjective (or sometimes named after an individual) and indicates or describes the member of the genus. For example, the species name for sugar maple is Acer saccharum and the species name for white oak is Quercus alba. Again, the genus followed by the specific epithet comprises a species. A species is a group of individuals that can be characterized by a set of identifiable characteristics that distinguishes them from other types; thus, all sugar maples (Acer saccharum) look more or less alike and can be differentiated from other maple species.
There are rules for writing a species name. The genus name is capitalized and either italicized or underlined. For example, the genus for maples is written as Acer (or Acer). The plural of genus is genera. The specific epithet is written in lower case and is italicized or underlined. For example, the Japanese maple is Acer palmatum (or Acer palmatum). Italics or underline designates the names are in a foreign language. The plural of species is species; the abbreviation for a species (singular) is sp. and the abbreviation for more than one species is spp. (plural). For example, one maple species is Acer sp. and more than one species is Acer spp. In paragraph style, after the first mention of a species, e.g., Acer palmatum, the following references to that species use a first letter abbreviation of the genus term, e.g., A. palmatum. Here is review example for the proper designation of a scientific plant name, Acer palmatum:
Species: Acer palmatum
Genus: Acer
Specific epithet: palmatum
Common name: red maple
Knowing the Latin meaning of the binomial can often help one remember the species name. For example, in the species Cornus florida, flowering dogwood, the genus Cornus means horn, referring to the very hard wood of this species. The specific epithet, florida, means flowering referring to the very showy flowers. Not all binomial names have meanings that are linked with tangible nouns or adjectives. For example, the genus Pinus (pine) refers to the name historically given to pine trees.
A scientific name and a common name may be learned with equal ease. We just need to make the association with the plant. Most people never really take a close look at the scientific name because it appears so foreign, hard to remember, impossible to pronounce, and of no help at all in remembering the plant. In fact, the Latin genus and species may be quite familiar to us and help more than we may think in making the plant-to-name association. Pronunciation does not need to be an undue burden either.
Pronouncing Latin names
Latin pronunciations of plant names have evolved from the classical form of the language to a more comfortable, modernized form. The following guide may help in understanding and using the pronunciations.
Table 1-2: Consonants
Letter | Sound | Example |
---|---|---|
C- | soft (as in city) when followed by E, I, Y, AE, or OE hard (as in call) when followed by A, O, U, AU, OI, or a consonant |
Cycas = SIGH-kus Coccinea - kok-SIN-ee-uh |
G- | soft (as in gem) when followed by E, I, Y, AE, or OE hard (as in go) when followed by A, O, U, AU, or OI |
Ginkgo = JINK-go |
CH- | always as K (as in chemist) unless part of a proper name | Chamaedorea = kam-ee-DOR-ee-uh Pachysandra = pak-ih-SAN-druh Veitchia = VEETCH-ee-uh (for English Nursery) |
Table 1-3: Vowels
Letter | Sound |
---|---|
A- | long as in fate (sometimes fat), short as in idea |
E- | long as in be; short as in bell |
I- | long as in pine (sometimes machine); short as in pin. Species names ending in -ii have the first pronounced as machine and the second as in side (-ii = ee-eye) |
O- | long as in note; short as in not |
U- | long as in rule; short as in up |
Y- | long as in type; short as in symbol |
Table 1-4: Dipthongs
Dipthong | Sound | Example |
---|---|---|
ae- | as in Caeser | dracaena = dra-SEE-nuh |
oe- | as in oenology | amoena - a-MEE-nuh |
au- | as in author | centaurea = cen-TAU-ree-uh |
eu- | as in neuter | leucothoe = loo-KOTH-oh-ee |
oi- | as in coin | deltoides = del-TOY-deez |
Accents: Final syllables are never accented. When the name has three or more syllables, the next to last is generally accented. If that syllable is very short (especially if a single vowel), the accent is commonly placed on the preceding syllable. Note: Common usage sometimes interferes with these rules, and some plant dictionaries may even disagree.
Gypsophila = jip-so-PHIL-uh rather than jip-SOPH-il-uh
Pittosporum = pit-oh-SPOR-um rather than pit-TOSS-pore-um
Araucaria = ar-au-CARE-ee-uh rather than ar-au-care-EE-uh
Examples:
Cordyline terminalis: kor-dih-LYE-nee ter-mih-NAY-lis
Kalachoe blossfeldiana: kal-an-KOH-ee bloss-fel-dee-AYN-uh
Sedum acre: SEE-dum AY-kree
Many Latin genus names have been adopted into our common name vocabularies, such as Aucuba, Begonia, Dieffenbachia, Draceana, Forsythia, Gladiolus, Petunia, and Philodendron. (They are not italicized or underlined when used as English common names, but still must be when including the Latin species.) Other times, the common name is a direct English version of the Latin genus, as in juniper from Juniperus, lily from Lilium, pine from Pinus, rose from Rosa, and spirea from Spiraea. For other genera, the name derivation is not as direct, but with repetition, it is equally easy to relate Acer as maples, Dianthus as pinks, Ilex as hollies, Ligustrum as privets, or Taxus as yews.
Looking at these Latin words may offer instant recognition or be challenging. Further help could really benefit our learning effort. One good reference is Botanical Latin (W.T. Stearn, 2004, Hafner Publishing Co., New York) is more for the taxonomist than the gardener. Another is A Dictionary of Botanical Terms (F.A. Swink, 1990, American Nurseryman Publishing Co.). A favorite is the soft-bound, pocket-size New Pronouncing Dictionary of Plant Names (rev. 2006). As the title says, both pronunciations and translations are provided for a wide array of genus and species names. It also may be obtained from American Nurseryman Publishing.
Plant Classifications Subordinate to a Species (Subspecies, Variety, Cultivar, Hybrid)
Plants of a particular species are not identical. They may have a different form, leaf size, flower color, growth rate. To appreciate this phenomenon, consider the wide variety of phenotypic (visual appearance as a result of DNA expression) traits that makes each one of us who we are: hair, eye, and skin color, height, hand size, and body type are a few traits that make each one of us unique. The same phenotypic variation occurs in plant populations. So, in a population of 1,000 seed-grown redbud trees (Cercis candensis), most plants will have the typical leaf size, flower color, and tree height. A small proportion will, however, have large leaves or small leaves, some have light pink flowers and some will have dark pink/purplish or even white flowers, some will have a showy yellow fall foliage color and others not, and so on. This variation is termed intra-specific (within a species) variation. The reason for this variation is sex. All offspring derived from seeds are the result of the mixing of male and female genetic material from each parent. In the case of plants, pollen grains (male) unite with an “egg” (female) to form a seed.
There are a few important nomenclature categories that are subordinate to a species. They are subspecies, variety, and cultivar.
Subspecies
A subspecies is a grouping within a species used to describe geographically isolated variants. It is a category above variety and is indicated by the abbreviation “subsp.” Not all species have subspecies.
An example of subsp. use is Hydrangea anomala subsp. petiolaris (climbing hydrangea). There is usually a wide range of intra-specific variation for numerous traits in a population of a seed-propagated species. In addition to obvious traits such as leaf and flower sizes and colors, vigor, plant form, and dwarfism, there may also be differences in less obvious traits, such as tolerances to external influences such as pests, low and high temperatures, and soil moisture aspects. You should have an appreciation for intraspecific (within a species) variation. Why? First, this wide degree of variation is necessary for plants to adapt to the dynamic nature of the environment. For example, the genetic capacity to produce small leaves may be advantageous in climates/environments where rainfall is relatively low since small leaves lose less water via transpiration (water vapor that exits leaf pores) than large leaves. Conversely, the genetic capacity to produce large leaves may be advantageous in climates/environments with ample rainfall to have a larger leaf surface to capture more sunlight and produce more sugars via photosynthesis. Secondly, having a keen eye for this variation may make you rich. You may discover an individual plant that has a particularly unique, attractive, and marketable trait. You can introduce, trademark, and perhaps patent this clone. New-to-the-trade plants are very popular and generate a lot of plant sales and profit.
Variety
A variety is a subpopulation of a species that has a distinctive trait that distinguishes it from the rest of the species and occurs in nature. Many species variety traits are inheritable, and succeeding seedling generations will express that trait; hence varieties are “true to seed.” True to seed refers to the phenomenon where a distinctive trait is usually inherited and expressed when propagated via seeds (sexual propagation). For example, most redbuds (Cercis canadensis) produce pinkish flowers in the spring, whereas the variety alba of redbud (Cercis canadensis var. alba) produces white flowers. Thus, seed from Cercis canadensis var. alba will result in most, but probably not all, of those seedlings ultimately producing white flowers. Another example, the common honeylocust, Gleditsia triacanthos, has very large thorns. The thornless subpopulation of this species is the variety inermis (meaning thornless; Gleditsia triacanthos var. inermis). As in the redbud example, most plants produced from seeds from Gleditsia triacanthos var. inermis will be thornless.
A variety is part of the scientific name and the word variety is abbreviated, var., and placed after the specific epithet and the italicized variety term follows var. Thus, the scientific name for redbud (usually pink-flowered) with white flowers is Cercis canadensis var. alba. Note that the var. term is lower case and not italicized. Here is an example for the proper designation of a plant variety name, Cercis canadensis var. alba
Genus: Cercis
Specific epithet: canandensis
Variety abbreviation: var.
Variety term: alba
Cultivar
Another species subclassification is a cultivar. The word cultivar is the shortened version of a cultivated variety, which is a variety that was developed or selected by humans. A cultivar is an assemblage of plants that has been selected for a particular attribute or combination of attributes and that is clearly distinct, uniform, and stable in these characteristics and that retains those characteristics when propagated. Most cultivars must be propagated asexually. The cultivar name is not in Latin. The first letter of each word (if more than one word) is upper case, and put in single quotes. For example, the purple-leaved cultivar of redbud is Cercis canadensis ‘Forest Pansy.’
Genus: Cercis
Specific epithet: canadensis
Cultivar: ‘Forest Pansy’
In some cases there are cultivars of a variety such as the thornless common honeylocust cultivar (‘Sunburst’) that has chartreuse new foliage. The name for this plant is Gleditsia triacanthos var. inermis ‘Sunburst’. There are three main distinctions between a cultivar and a variety.
1) Whereas a variety has a natural connotation, a cultivar has a commercial connotation (used in horticultural commerce).
2) Most woody plant cultivars are not discovered as a subpopulation, but are the result of a bud sport (bud mutation), a seedling plant with a distinctive trait, a witches’ broom (plant tissue that usually arises from a microorganism invading plant tissue and causing dwarfism), or human-mediated mutagenic alterations. In the case of a bud sport, when a bud (small package of embryonic tissue which becomes a leaf, stem, or flower) is forming, a mutation occurs that can give rise to a distinctive trait.
3) To introduce the third distinction, you should know that grasses and some annuals (non-woody plants) are generally “true-to-seed.” This means that the offspring of true-to-seed species will exhibit the characteristics of the parents (due to “well behaved” gene mixing during reproduction). This is why we can plant seed of corn, turfgrass species, and marigolds, for example, and get plants that will exhibit the cultivar characteristics of those mother plants. In contrast, most woody plant cultivars are not true-to-seed (due to a more random mixing of genes during reproduction). Hence, woody plants must be asexually propagated (e.g., cuttings, grafting, layering, and micro-propagation) to maintain their cultivar characteristic. For example, if you plant a seed from a ‘Delicious’ apple, you will not get an apple tree that produces fruit with the characteristic shape and sweet taste traits of a ‘Delicious’ apple; you will get an apple that may be round and bitter since the genetic makeup of a ‘Delicious’ apple was not preserved during seed formation. Accordingly, seedlings from a ‘Sunburst’ honeylocust (Gleditsia triacanthos var. inermis ‘Sunburst’) will not have the chartreuse new foliage characteristic, but most of them will be thornless. This is because the cultivar characteristic is usually not true-to-seed for woody plants, whereas the variety characteristic (thornlessness) is usually true-to-seed. Thus, to propagate a woody plant cultivar with a distinctive trait, one must clone the plant by an asexual propagation technique (e.g., cuttings or grafting). The distinction between variety and cultivar gets fuzzy because woody plant varieties are commonly sold in the horticultural trade (sometimes as cultivars), and there are a very few woody plant cultivars that are true-to-seed.
Cultivar traits and trait stability
There are numerous cultivar traits such as variegated (colors other than the species’ characteristic green) leaves, plant size/form, flower color/size/petal number/fragrance, fruit size/color, disease resistance, and growth rate. For example, Acer platanoides ‘Drummondii’ (Drummondii Norway maple) has variegated foliage, leaves with broad white edges. If a cultivar partly or totally ceases to express its cultivar characteristic trait and produces an appearance that is the same as the species, then that cultivar has reverted (reversion) to the species characteristics. Portions of an Acer platanoides ‘Drummondii’ tree characteristically revert to the all green leaves. Thus when reversion occurs, the “genetic switch” to control the expression of the cultivar trait (leaf variegation in this case) is turned off, and the “genetic switch” controlling the expression of the normal species leaf color is turned on. Some cultivar traits are very stable and rarely revert to the species form, some cultivars revert occasionally, and some cultivars revert commonly. Commonly, and in some cases occasionally, reverting cultivars make poor landscape plants since they do not retain their unique cultivar trait and plants have to be pruned to remove the reversions. Of course, if a reversion occurs in the upper portion of a tree, then the reverted branch would be difficult to remove.
The popular dwarf Alberta spruce (Picea abies ‘Conica’), a dwarf, slow-growing, compact, cone-shaped form of white spruce, will occasionally revert to the species characteristics (medium tree with a faster growth rate, much larger needles and wide-spaced branches). In this case the branch sport (reversion) exhibiting the species characteristics will ultimately outgrow the plant and the result will be a very awkward looking specimen. To avoid this, the reversion should be cut out as soon as possible to maintain the character of the cultivar.
Taxon
A taxon, an abbreviation of “taxonomic group” or “taxonomic unit,” is any taxonomic group/category; the plural is taxa. For example, a genus is a taxon, a cultivar is a taxon, and a genus, species, and cultivars are three taxa.
Trademark
The primary role of a trademark is to indicate the source of goods and is not intended to label an individual product or cultivar. For example, the very popular Wave™ petunia series includes Wave™ Blue, Wave™ Misty Lilac, and Wave™ Purple. Cultivar names are the domain of the public, and therefore cannot be trademarked. There are two types of trademarks. The common use trademark is designated with the superscript TM, such as in the previously mentioned Wave™ petunia series. Common business use of trademark name grants a business the rights to that name. The second trademark type is a registered trademark in which the trademark is registered by the United States Patent and Trademark Office. A registered trademark is designated by a superscript ®, is valid for 10 years, and is renewable. By ascribing a trademark name (either type) to a plant, a company has the sole right to selling a plant by that trademark name. However, another company can sell that plant by its cultivar name.
Unfortunately, some plant catalogs and text books incorrectly put trademark names in single quotes (cultivar designation). Such errors result in considerable confusion between cultivar and trademark names. Due to the proliferation of new plant introductions, many of which have been trademarked, many plants are known by their trademark name, and some of these trademark names are erroneously put in single quotes.
Plant patent
A plant patent gives the patent owner the sole right to reproduce, sell, or use an asexually propagated plant. A patent grant lasts for 20 years from the date of filing the patent application, and is not renewable. Patented plants are generally trademarked. Patented plants have the letters PP (plant patent) next to their name. Plants in the process of being patented have the acronym PPAF (plant patent applied for) which carries no legal validity.
Hybrids
A hybrid is the result of a sexual cross, transfer of pollen (sperm) of one plant to the pistil (contains ovary/egg) of another plant, between two or more plants that are somewhat related. Most of the time, a hybrid is a cross between two different varieties of the same species. Occasionally two different species of the same genus are crossed. The resulting plant is called an interspecific (between species) hybrid. For example, two Abelia species, A. chinensis and A. uniflora were crossed, and the relatively common landscape shrub A. ×grandiflora (glossy abelia) was produced.
Genus: Abelia
Multiplication symbol: x (represents a genetic cross)
Specific epithet (no space between x and specific epithet): grandiflora
As seen in this example, an interspecific hybrid is designated by placing a multiplication symbol (or lowercase x) immediately (no space) before the specific epithet. An intergeneric (between genera) hybrid is a cross between two genera and is a very rare occurrence. An intergeneric hybrid is designated by placing the × symbol immediately in front of the genus, for example, ×Sycoparrotia semideciuda which is a hybrid between Parrotia persica and Sycopsis sinensis.
What Do Those Words Mean?
This short list of Latin words used in plant names offers a glimpse of the information they provide. Adjectives here are presented in the masculine form to go with a masculine noun (genus). The endings would differ for a feminine or neuter genus, but the root portion would stay the same.
Designating Plant Habitat
aquaticus = in water
arvensis = in fields
maritimus = by the sea
palustris = in swamps
pratensis = in meadows
sativus = cultivated
sylvestris, sylvaticus = in woods
Designating Plant Geography
americanus = Americas
australis = southern
borealis = northern
canadensis = Canada (N. US)
carolinianus = Carolinas
chinensis, sinensis = China
occidentalis = western (New World)
virginianus = Virginias
orientalis = eastern (Old World)
Designating Plant Attributes
annus = annual
officianales = medicinal
communis, vulgaris = common
parennes = perennial
pulchellus = beautiful
rugosus = wrinkled
setaceus = bristle-like
spectabilis = handsome, showy
vernus = spring flowering
Designating Plant Appearance
gracilis = graceful, slender
humilus = low
procumbens = trailing
pubescens = downy hair surface
pumilus, nanus = dwarf
repans, reptans = creeping
scandens = climbing
tuberosus = forming tubers
Designating Plant Parts
carpus = fruit
caulis = stem
florus, anthos = flower
folium, phyllon = leaf
Designating Color
albus = white
atropurpureus = dark purple
aureus = golden
bicolor = of two colors
coccineus = scarlet
concolor = same color both sides
discolor = different color each side
flavus, luteus = yellow
glaucus = whitish with a bloom
niger = black
ruber = red
sanguineus = blood-red
variegatus = variegated
viridis = green
Designating the Collector (occasionally capitalized)
fortunei = from Fortune
halliana = from Hall
sargentii = from Sargent
thunbergii = from Thunberg
Numerical Prefixes
uni-, mono- = one
bi-, di- = two
tri– = three
quadri-, tetra- = four
quinque-, penta- = five
multi– = many
a- = without, lacking
Descriptive Prefixes
albi-, leuco– = white
alterni– = alternate
angusti– = narrow
brevi– = short
grandi– = large
hetero– = differing
lati– = broad
longi– = long
micro– = small
macro– = large, long
rotundi– = round
semper– = always
Identification With an Analytical Key
The ability to identify plants is something like learning people’s names. When you are introduced to someone by name, you may concentrate on associating the name with particular features of that person, whether they are facial or other physical features. If you meet a person only once, you will probably soon forget who the person is. However, after you have been associated with a person for a long time and have called him or her by name a number of times, you will then be able to identify that person when you see him/her anywhere. If you do not see him/her for a few years, you may find that you have forgotten the name, but it will only take a short reminder to re-familiarize yourself with that person. The same process is involved in identifying plants. The significant difference between identifying plants and identifying people is that plants can’t tell you who they are. Consequently, various guides have been prepared to assist in determining the identity of various plants. The bibliography at the end of this section lists several of these manuals.
The identification of an unknown plant usually requires an analytical key, or identification tool, which is a part of most identification manuals. These keys list plant features, such as leaf arrangement, leaf shape, leaf color and hairiness, various twig features, and many other identifying characteristics that are evident at various times of the year. Usually the keys are based on vegetative features, even though the first separation of plants by species was based on differences in sexual structures. Unfortunately, the flowering stages do not last long enough to use this as a general means of identifying plants.
The use of a key is a step-by-step process of elimination, beginning with the most general characteristics (for example, evergreen vs. deciduous) and progressing to the most specific characteristics. Most manuals will begin with instructions for their use, as well as definitions of the various characteristics. The terms used in the definitions can differ slightly between manuals, so review of this section is important.
Additional Resources
- “Name that Plant – The Misuse of Trademarks in Horticulture
- Evert, Ray F. and Eichhorn, Susan E. Raven Biology of Plants. 2012. W. H. Freeman.
- For more on absorption: “How Vegetable Plant Roots Absorb Nutrients” by Mosaic Crop Nutrition
References
“Plant Cell Structure and Function” section adapted from Evert, Ray F. and Eichhorn, Susan E. Raven Biology of Plants. 2012. W. H. Freeman.
Leaf blade shapes adapted from Colorado State University Extension Master Gardener Training, “CMG GardenNotes #134: Plant Structures: Leaves.” 2017.
Attributions
- Susan Dudley, Norfolk Extension Master Gardener (2021 reviser)
- Wendy Silverman, New River Valley Extension Master Gardener Coordinator (2021 reviser)
- Lisa Sanderson, Extension Agent, Agriculture and Natural Resources (2015 reviser)
- Stuart Sutphin, Extension Agent, Agriculture and Natural Resources (2015 reviser)
- J. Christopher Ludwig, Chief Biologist, Virginia Department of Conservation and Recreation (2015 reviewer)
- Alan McDaniel, Extension Specialist, Horticulture (2009 reviser)
- Thank you to Dr. Eric Beers, School of Plant and Environmental Sciences, Virginia Tech
Image Attributions
- Figure 1-1: Plant cell diagram. Johnson, Devon. 2022. CC BY-NC-SA 4.0. Includes CNX OpenStax. 2016. Figure 04 03 01b.png CC BY-NC-SA 4.0. From WikimediaCommons
- Figure 1-2: Principal parts of a vascular plant. Johnson, Devon. 2022. CC BY-NC-SA 4.0. Adapted from “1–9 A modern vascular plant” in Raven Biology of Plants. 2012. by Eichhorn, Susan E., and Evert, Ray F. Originally from W. Troll. 1937. Vergleichende Morphologie der Hoheren Pflanzen, vol. 1, pt. 1, Verlage von Gebrüder Borntraeger, Berlin.
- Figure 1-3: Three examples of organization of vascular system in stem, shown in cross-section. Johnson, Devon. 2022. CC BY-NC-SA 4.0. Adapted from “25–8 Three basic types of organization in the primary structure of stems, as seen in transverse section” in Raven Biology of Plants. 2012. by Eichhorn, Susan E., and Evert, Ray F.
- Figure 1-4: Stem nodes. Grey, Kindred. 2022. CC BY-NC-SA 4.0.
- Figure 1-5: Spur. Grey, Kindred. 2022. CC BY-NC-SA 4.0.
- Figure 1-6: Diagram of a crown and runner. Grey, Kindred. 2022. CC BY-NC-SA 4.0.
- Figure 1-7: Image of a stolon. Grey, Kindred. 2022. CC BY-NC-SA 4.0.
- Figure 1-8: Image of a rhizome. Grey, Kindred. 2022. CC BY-NC-SA 4.0.
- Figure 1-9: Image of a bulb. Grey, Kindred. 2022. CC BY-NC-SA 4.0.
- Figure 1-10: Image of a corm. Grey, Kindred. 2022. CC BY-NC-SA 4.0.
- Figure 1-11: Image of a tuberous stem. Johnson, Devon. 2022. CC BY-NC-SA 4.0.
- Figure 1-12: Tuberous root of dahlia. Johnson, Devon. 2022. CC BY-NC-SA 4.0.
- Figure 1-13: Types of vines. Johnson, Devon. 2022. CC BY-NC-SA 4.0.
- Figure 1-14: Broadleaf diagram. Grey, Kindred. 2022. CC BY-NC-SA 4.0.
- Figure 1-15: Conifer leaf diagram. Grey, Kindred. 2022. CC BY-NC-SA 4.0.
- Figure 1-16: Cross section of a leaf. Johnson, Devon. 2022. CC BY-NC-SA 4.0.
- Figure 1-17: Images of stoma. CNX OpenStax. 2016. Figure_30_02_05abc.jpg. CC BY-NC-SA 4.0. From WikimediaCommons
- Figure 1-18: Simple leaf. Grey, Kindred. 2022. CC BY-NC-SA 4.0.
- Figure 1-19: Three examples of compound leaves; palmate, pinnate, and double pinnate compound. Grey, Kindred. 2022. CC BY-NC-SA 4.0.
- Figure 1-20: Types of leaf arrangements. Grey, Kindred. 2022. CC BY-NC-SA 4.0.
- Figure 1-21: Three examples of venation in leaves; parallel, pinnate, and palmate. Johnson, Devon. 2022. CC BY-NC-SA 4.0.
- Figure 1-22: Leaf blade shapes. Grey, Kindred. 2022. CC BY-NC-SA 4.0.
- Figure 1-23: Leaf apex shapes. Grey, Kindred. 2022. CC BY-NC-SA 4.0.
- Figure 1-24: Leaf base shapes. Grey, Kindred. 2022. CC BY-NC-SA 4.0.
- Figure 1-25: Leaf margin shapes. Grey, Kindred. 2022. CC BY-NC-SA 4.0.
- Figure 1-26: Twig diagram. Grey, Kindred. 2022. CC BY-NC-SA 4.0.
- Figure 1-27: Leaf and buds of Elm. Grey, Kindred. 2022. CC BY-NC-SA 4.0.
- Figure 1-28: (A) Cross section of monocot Smilax spp. root… Berkshire Community College Bioscience Image Library. Flickr. 2014. Public Domain. and “Young Herbaceous Dicot Root: Ranunculus.” Berkshire Community College Bioscience Image Library. Flickr. 2014 Public Domain.
- Figure 1-29: Fibrous root system of Aristida purpurea. Includes “Fig. 43 — Wire grass (Aristida purpurea)” and ” Fig. 46.— A loco weed (Aragallus lambertii)” by Weaver, John E. 1926. Root Development of Field Crops. McGraw-Hill, New York. Public Domain. Accessible here.
- Figure 1-30: Apical meristem of Allium spp. Berkshire Community College Bioscience Image Library. Flickr. Public Domain.
- Figure 1-31: Zones of elongation. Johnson, Devon. 2022. CC BY-NC-SA 4.0.
- Figure 1-32: Parts of a flower. Grey, Kindred. 2022. CC BY-NC-SA 4.0.
- Figure 1-33: Racemose. Grey, Kindred. 2022. CC BY-NC-SA 4.0.
- Figure 1-34 Cyme. Grey, Kindred. 2022. CC BY-NC-SA 4.0.
- Figure 1-35: Types of fruit. Johnson, Devon. 2022. CC BY-NC-SA 4.0. Includes Isolated Pineapple by Schwarzenarzisse on Pixabay and Raspberry Fruit by l_cwojdzinski on Pixabay and Blue Jay Eating Peanut by edbo23 on Pixabay.
- Figure 1-36: Parts of a seed. Grey, Kindred. 2022. CC BY-NC-SA 4.0. Adapted from Master Gardener Training Handbook, Virginia Cooperative Extension, 2018.
- Figure 1-37: Germination of a dicot bean seed and monocot onion seed. Johnson, Devon. 2022. CC BY-NC-SA 4.0. Adapted from Master Gardener Training Handbook, Virginia Cooperative Extension, 2018.
- Figure 1-38: The bounty from the Resiliency Garden at Fort Belvoir. Wellington, Kathleen. GSEMG: Wellness Circle and USO Resiliency Gardens. CC BY-NC-SA 4.0.
- Figure 1-39: Photosynthesis. Johnson, Devon. 2022. Adapted from Photosynthesis_en.svg by At09kg, Wattcle, and Nefronus. 2016. WikimediaCommons. CC BY-NC-SA 4.0.
- Figure 1-40: Guard cells surrounding open stoma in epidermis of succulent xerophyte leaf. Berkshire Community College Bioscience Image Library form Flickr. 2014. Public Domain.
- Figure 1-41: Cross section of a leaf (dots represent relative humidity). CC BY-NC-SA 4.0. from Master Gardener Training Handbook, Virginia Cooperative Extension, 2018.
Seed-bearing vascular plants that produce exposed seeds, or ovules, which are usually borne in cones
Flowering plants that produce seeds enclosed in a fruit
Flowering plants with seeds that contain a pair of embryonic leaves (cotyledons). Most of the broadleaf herbs, shrubs, and trees are dicots.
Grass and grass-like flowering plants with seeds that typically contain only one embryonic leaf
A rigid, structural layer outside the cell membrane in cells of plants, fungi, and some other organisms
Organelle that contains the genetic information for the organism and controls the activities of the cell
A large liquid-filled cavity within a cell
Sites of photosynthesis within cells that contain chlorophylls and carotenoid pigments
Transport tissue in vascular plants, transports water from roots to stems and leaves (also transports nutrients)
Transport tissue in vascular plants, transports the soluble organic compounds made during photosynthesis to the rest of the plant in a process called translocation
Part of the transport system in vascular plants that includes the xylem, phloem, and other tissues
The part of the stem where one or more leaves are attached
Section of the stem between two successive nodes
Small package of partially preformed tissue which becomes leaves/stems or flowers
A but that contains partially preformed flower tissue
A bud that contains partially preformed leaf and stem tissue
Region of compressed stem tissue from which new shoots are produced, generally found near the surface of the soil
A region of compressed stem tissue from which new shoots are produced
Horizontal stem that is fleshy or semi-woody and lies along the top of the ground
A specialized stem that grows on the soil surface and forms a new plant at one or more of its nodes. A type of stolon.
Specialized stem that grows underground and sends out roots and shoots from nodes
Shortened, compressed, underground stems surrounded by fleshy scales (leaves) that envelop a central bud located at the tip of the stem
A solid, swollen stem whose scales have been reduced to a dry, leaf-like covering. They have shapes similar to bulbs, but do not contain fleshy scales.
Enlarged portion of an underground stem. The tuber, like other stems, has nodes that produce buds.
Modified lateral roots that are enlarged to function as an underground storage organ. Found in dahlia and sweet potato.
A young stem with leaves present
A stem that is less than one year old and has no leaves since it is still in the winter-dormant stage
A stem that is more than one year old and typically has lateral stems
A main stem of a woody plant
Perennial woody plants that have one or several main stems, and usually are less than 12 feet tall at maturity.
A plant that develops long, trailing stems that grow along the ground unless they are supported by another plant or structure
A stem that has a relatively large pith (the central, strength-giving tissue of stem) and usually lives only one or two years
Plants that pass through their entire life cycle from seed germination to seed production in one growing season, then die
Plants that start from seeds and produce vegetative structures and food storage organs the first season and flowers, fruit, and seeds the second season
Plants that live for many years. May be herbaceous or, if significant xylem develops in the stem and the top persists, may be classified as woody.
Expanded, thin structure on either side of the midrib of a leaf. The blade is usually the largest and most conspicuous part of a leaf.
Stalk that supports the leaf blade
Protective film covering the outermost layer of epidermis in leaves, some shoots and some other plant organs.
Openings in leaves that allow passage of water and gasses into and out of the leaf. Singular: stoma
Epidermal cells located around a stoma that help regulate gas exchange by opening and closing
The small, leathery, protective leaves that enclose and protect buds, found on rhizomes and are
Modified leaves that are found on the embryonic plant and commonly serve as storage organs
Specialized modified leaves that protect the plant
Serve as food storage organs, found on bulbous plants and succulents
Modified leaf or scale with a flower or flower cluster in its axil. Often brightly colored, as in poinsettias.
Leaves with a leaf blade that is a single continuous unit
Leaf shape in which leaflets form and radiate from a single point of attachment
Leaf shape in which leaflets are attached along an extension of the petiole
A circular arrangement of leaves, usually near the soil (for example, dandelion)
Leaf arrangement where leaves are positioned across the stem from each other, two leaves at each node.
Leaf arrangement where leaves are arranged in circles along the stem.
Leaf venation pattern in which numerous veins that run essentially parallel to each other and are connected laterally by minute, straight veinlets
Leaf venation pattern in which veins branch from the main rib(s), then subdivide into finer veinlets which then unite in a complicated network
Bud composed of a short stem with embryonic leaves
Buds located at the apex of a stem
Buds borne on the sides of a stem
Buds arising in the leaf axils
Buds arising at sites other than in the terminal or axillary position
Primary root, first organ to appear when a seed germinates
Formed when the primary root continues to elongate downward into the soil and becomes the central and most important feature of the root system, with a somewhat limited amount of secondary branching.
Side or branch root that arises from another root. Also called a secondary root.
Root system in which the primary root ceases to elongate, leading to the development of numerous lateral roots which branch repeatedly and form the feeding root system of the plant
Underground storage organ
An area of cell division and growth Cells in the meristem can develop into all other tissues and organs found in plants.
Projects of root epidermal cells, important in absorption of nutrients, plant anchorage, and more
Outermost tip of the root, consists of cells that are sloughed off as the root grows through the soil
Small, green, leaf-like structures on the base of the flower that protect the flower bud
The sepals of a flower
Modified leaves, typically brightly colored, segments of a flower’s corolla
The petals of a flower
The female part of the plant that consists of the stigma, style, and ovary
The male reproductive organ. It consists of a pollen sac (anther) and a long, supporting filament
A flower with a stamen, pistils, petals, and sepals
A flower with functional stamens and pistils
Flowers are those that possess a functional pistil(s), but lack stamens
Flowers that contain stamens, but no pistils
Plants with male and female reproductive organs on separate individuals
Plants with male and female reproductive organs on the same plant
Fruits that develop from a single ovary
Fruits that come from a single flower with many ovaries
Fruits derived from a tight cluster of separate, independent flowers borne on a single structure
Built-in food storage supply in a seed
The portion of a seedling between the radicle and the first leaf-like structure
Embryonic shoot
A small pore located in a seed's seedcoat that allows water absorption and gas exchange
Ability of plants to manufacture their own food
Process by which green plants and some other organisms use sunlight to synthesize foods from carbon dioxide and water. Generally involves the green pigment chlorophyll (in green plants) and generates oxygen as a byproduct.
Turgidity (swelling) and resulting rigidity of plant cells or tissues, typically from the absorption of fluid
Process by which a plant loses water, primarily from leaf stomata.
Process by which plants use the sugars produced during photosynthesis (plus oxygen) to produce energy
Process by which substances, particularly water and minerals, are moved into the plant. This occurs mainly through the roots in the tip region where root hairs are present, but it may also occur through leaf surfaces.
Plants that form their flowers only when the day length is less than about 12 hours in duration.
Plants form flowers only when day lengths exceed 12 hours (short nights).
Plants that form flowers regardless of day length
flower prematurely
The daily range of temperatures a plant is exposed to
The ratio of water vapor in the air to the amount of water the air could hold at a given temperature and pressure, expressed as a percent
The needs and uses of the basic chemical elements in the plant
When materials for plant nutrition are supplied to the environment around the plant
Chemical elements required in large amounts for plant growth and development. These are: Carbon (C), Hydrogen (H), Oxygen (O), Nitrogen (N), Phosphorus (P), and Potassium (K), Calcium (Ca), Magnesium (Mg), and Sulfur (S).
Chemical elements required in small amounts for plant growth and development. These are: Iron (Fe), Zinc (Zn), Manganese (Mn), Boron (B), Copper (Cu), Molybdenum (Mo), Chlorine (Cl)
A group of somewhat closely related individuals (a group name) comprising one or more species
A group of individuals that can be characterized by a set of identifiable characteristics that distinguishes them from other types
Variety bred by people to have desired traits that are reproduced in each new generation (usually through asexual propagation)
A genetic cross of two different plants, usually from two different varieties of the same species
System of naming organisms in which the name is composed of two terms
The second word of the Latin binomial that usually functions as an adjective (or sometimes named after an individual) and indicates or describes the member of the genus
Visual appearance as a result of DNA expression
Variation within a species
A grouping within a species used to describe geographically isolated variants
A subpopulation of a species that has a distinctive trait that distinguishes it from the rest of the species and occurs in nature
Any taxonomic group/category
A cross between different species of the same genus
Cross between two genera (a very rare occurrence)