18 Cytoskeleton
Learning Objectives
- Describe the traits and functions of the various cytoskeletal elements (microtubules, microfilaments, and intermediate filaments).
- Elucidate the general mechanism by which molecular motors move along cytoskeletal elements.
- Identify the molecular motors (dyneins, kinesins, and myosins) that partner with various cytoskeletal elements.
- Describe microtubule organizing centers, and their structures and functions.
- Explain the dynamic properties of cytoskeletal elements and how this relates to their construction and functioning.
- Elucidate the structure and function of cilia and flagella, and their differences and similarities.
- Elucidate the existence and importance of nonmuscle motility.
Key Takeaways
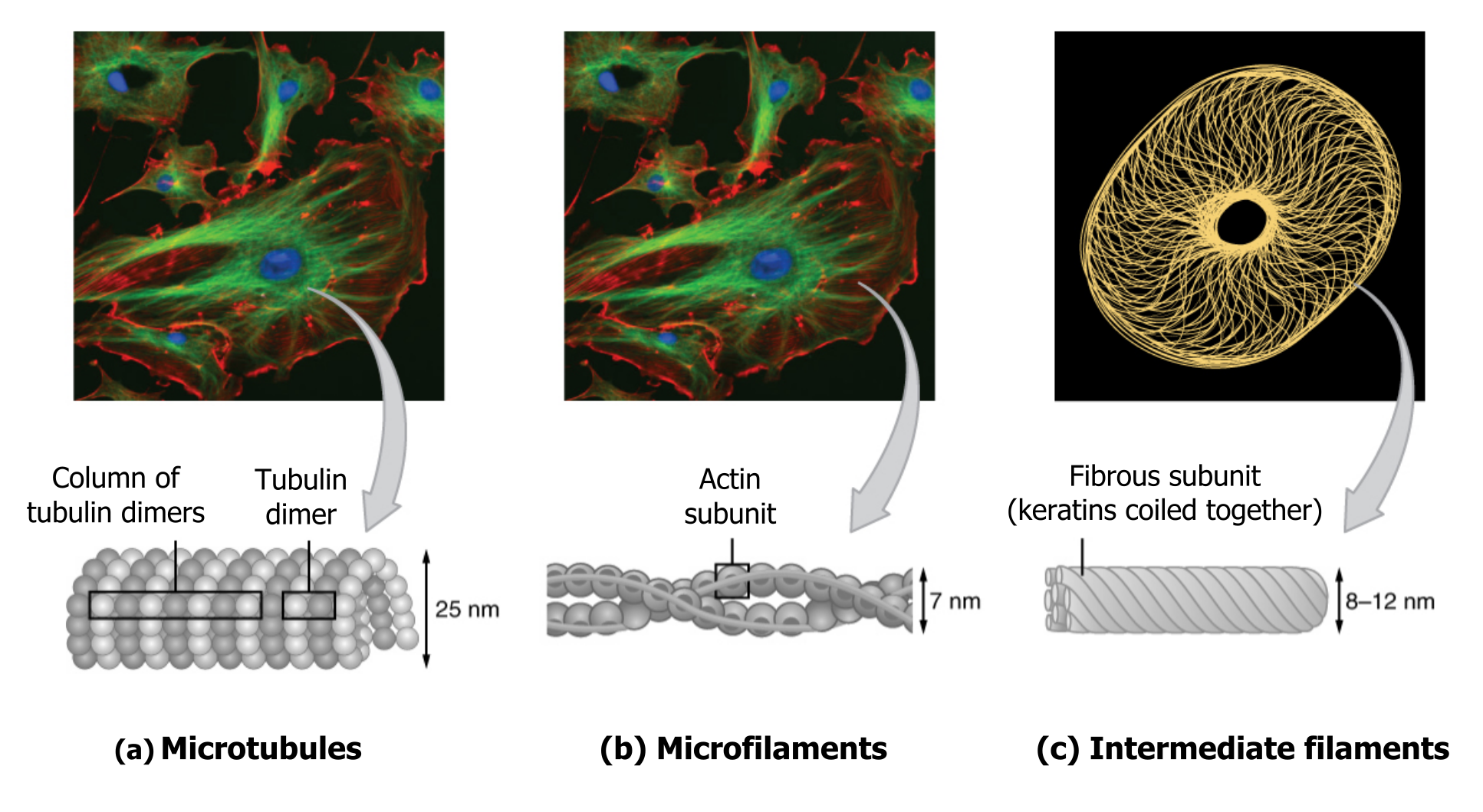
If you were to remove all the organelles from a cell, would the plasma membrane and the cytoplasm be the only components left? No. Within the cytoplasm, there would still be ions and organic molecules, plus a network of protein fibers that help maintain the cellʼs shape, secure some organelles in specific positions, allow cytoplasm and vesicles to move within the cell, and enable cells within multicellular organisms to move. Collectively, scientists call this network of protein fibers the cytoskeleton.
18.1 The Cytoskeleton
There are three types of fibers within the cytoskeleton:
- Microfilaments,
- Intermediate filaments, and
- Microtubules.
The collection of these fibers plays key roles in structure and support, intracellular transport, contractility and motility, as well as spacial organization (figure 18.2).
Microfilaments thicken the cortex around the cellʼs inner edge. Like rubber bands, they resist tension. There are microtubules in the cellʼs interior where they maintain their shape by resisting compressive forces. There are intermediate filaments throughout the cell that hold organelles in place.
Microfilaments
Of the three types of protein fibers in the cytoskeleton, microfilaments are the narrowest. They function in cellular movement, have a diameter of about 7 to 8 nm, and are comprised of two globular protein intertwined strands, which we call actin (figure 18.3). For this reason, we also call microfilaments actin filaments.
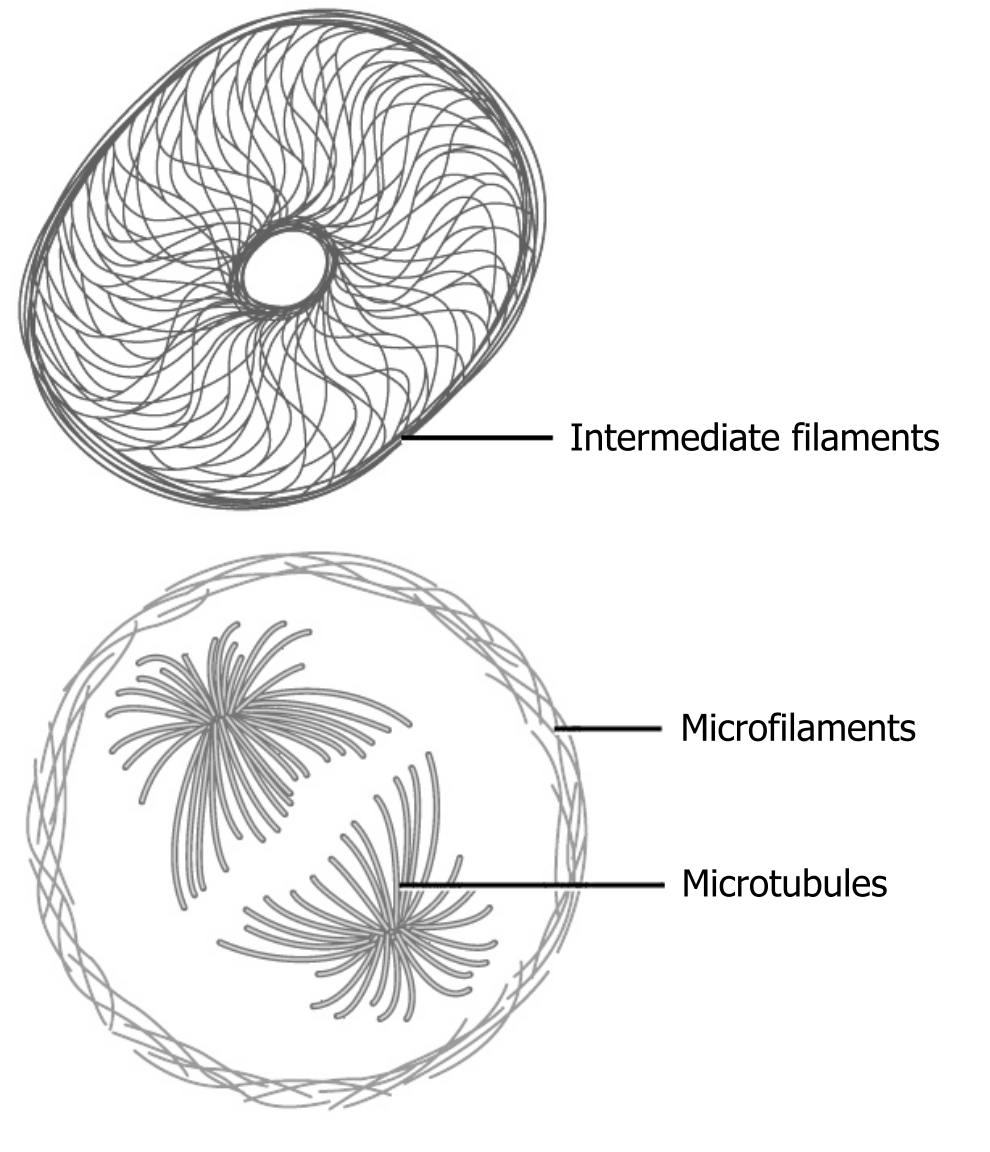
ATP powers actin to assemble its filamentous form, which serves as a track for the movement of a motor protein we call myosin. This enables actin to engage in cellular events requiring motion, such as cell division in eukaryotic cells. Actin and myosin are plentiful in muscle cells.
Microfilaments also provide some rigidity and shape to the cell. They can depolymerize (disassemble) and reform quickly, thus enabling a cell to change its shape and move.
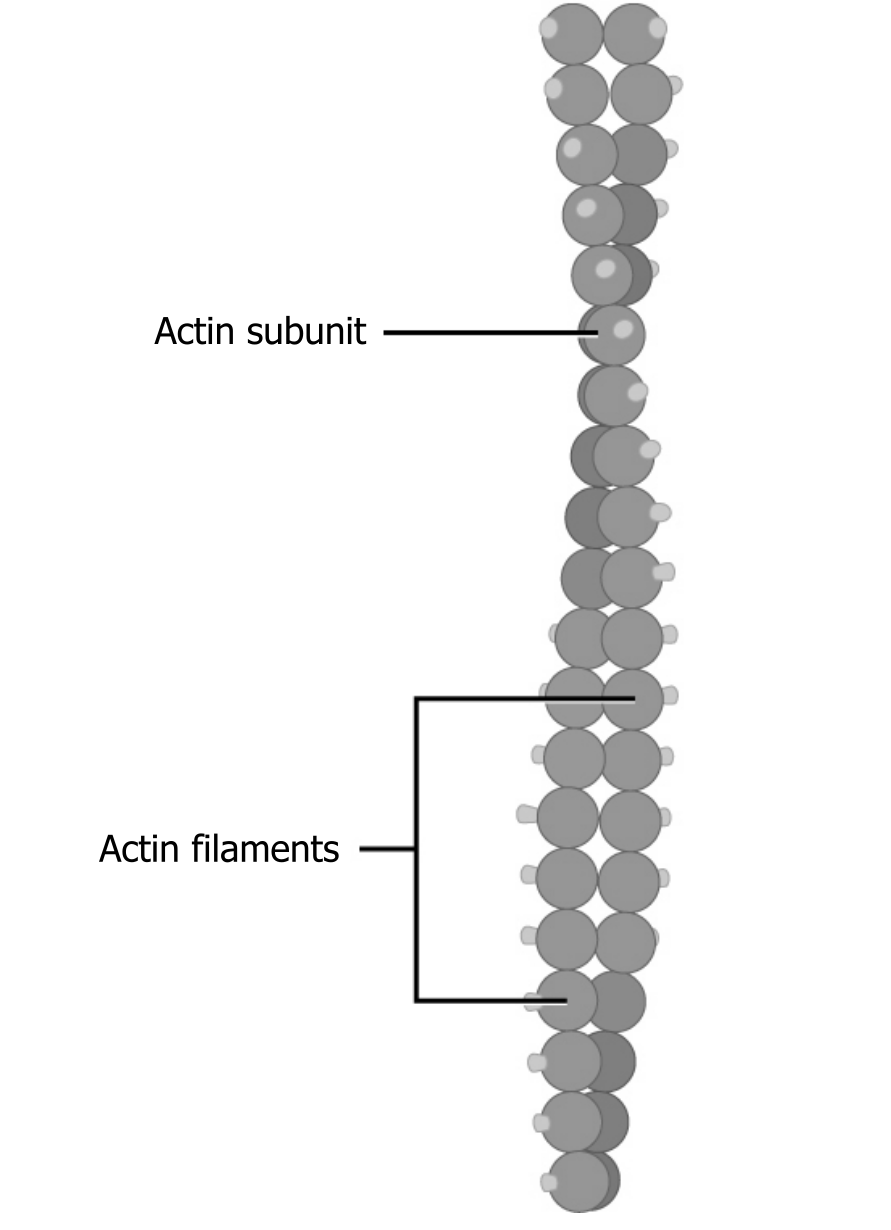
Intermediate filaments
Several strands of fibrous proteins that are wound together comprise intermediate filaments (figure 18.4). These cytoskeleton elements get their name from the fact that their diameter, 10 to 12 nm, is between those of microfilaments and microtubules.
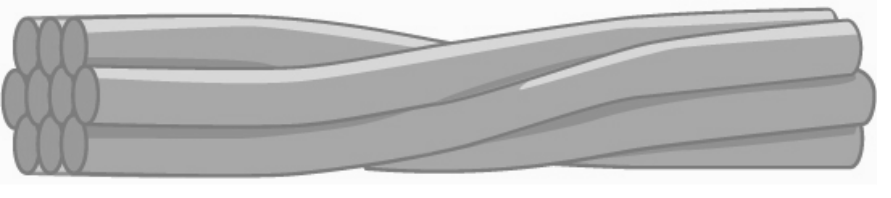
Intermediate filaments have no role in cell movement. Their function is purely structural. They bear tension, thus maintaining the cellʼs shape, and anchor the nucleus and other organelles in place (figure 18.1).
The intermediate filaments are the most diverse group of cytoskeletal elements. They are unbranched and rope-like with long fibrous subunits. There is no polarity associated with their assembly. Intermediate filaments are classified by their location and function. The table below summarizes various types of intermediate filaments.
Class | Protein type | Location | Function |
---|---|---|---|
1 & 2 | Keratins | Epithelial cells | Structural support, stretching |
3 | Vimentin | Fibroblasts/Epithelial cells | Cage-like structure around the nucleus |
Desmin | Muscle cells | Links myofibrils together | |
Glial fibrillary protein (GFAP) | Glial cells | Structural support | |
4 | Neurofilaments | Neurons | Support for axon and dendrites |
5 | Lamins | Nucleus | Support for inner surface of the nuclear membrane |
Table 18.1: Proteins and their functions.
Microtubules
As their name implies, microtubules are small hollow tubes. With a diameter of about 25 nm, microtubules are cytoskeletonsʼ widest components. They help the cell resist compression, provide a track along which vesicles move through the cell, and pull replicated chromosomes to opposite ends of a dividing cell (figure 18.5).
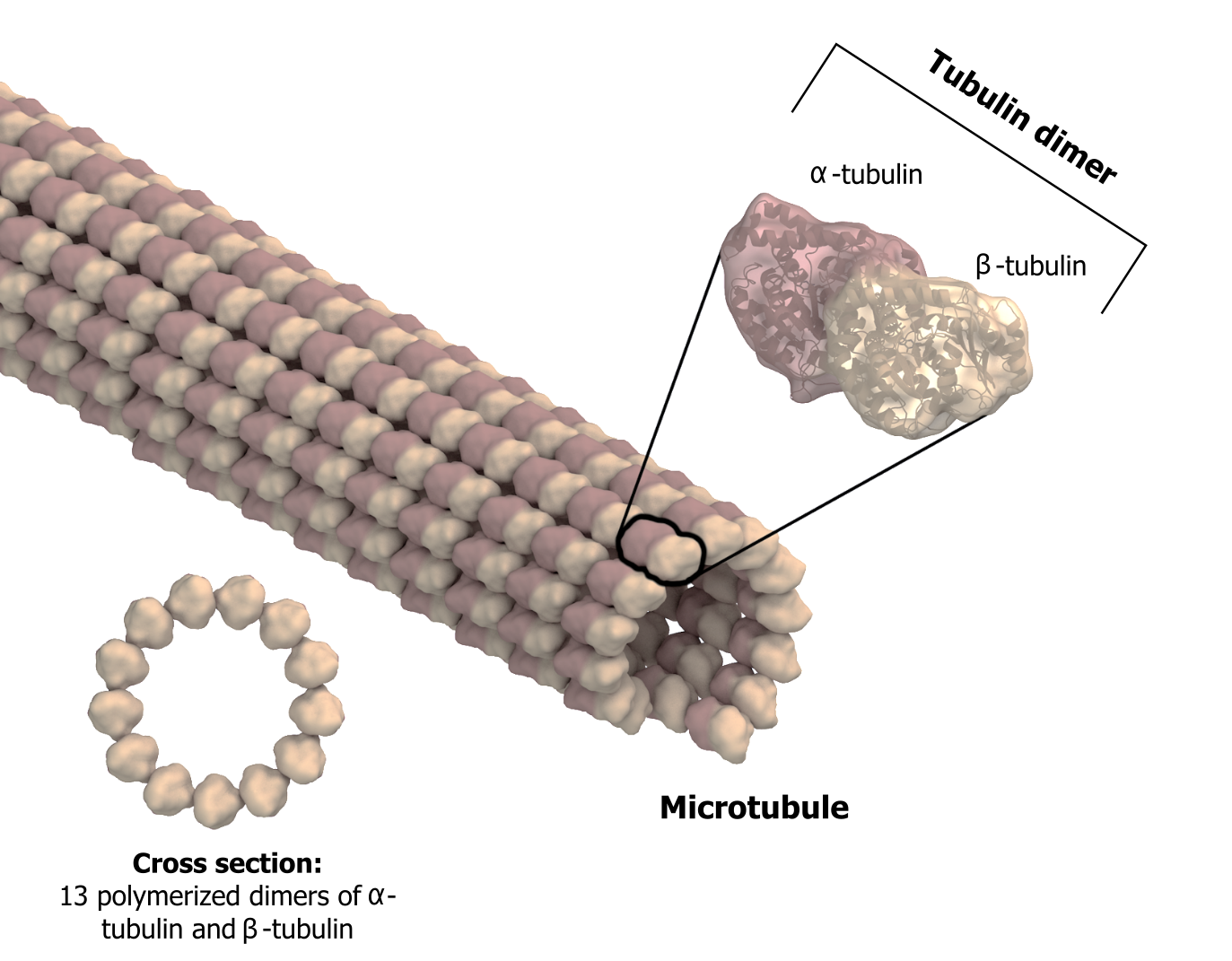
Like microfilaments, microtubules can disassemble and reform quickly using GTP. The tube is formed from polymerized dimers of α-tubulin and β-tubulin, two globular proteins. These proteins form long chains that comprise the microtubuleʼs walls. The assembly is slow and occurs from the plus end, which is designated by a row of β-tubulin. Disassembly can occur rapidly at the plus end. (Note the minus end has a row of α-tubulin.)
Microtubules are also the structural elements of flagella, cilia, and centrioles (the latter are the centrosomeʼs two perpendicular bodies). In animal cells, the centrosome is the microtubule-organizing center.
Flagella and cilia
The flagella (singular = flagellum) are long, hair-like structures that extend from the plasma membrane and enable an entire cell to move. When present, the cell has just one flagellum or a few flagella.
However, when cilia (singular = cilium) are present, many of them extend along the plasma membraneʼs entire surface. They are short, hair-like structures that move entire cells (such as paramecia) or substances along the cellʼs outer surface (for example, the cilia of cells lining the Fallopian tubes that move the ovum toward the uterus, or cilia lining the cells of the respiratory tract that trap particulate matter and move it toward your nostrils).
Despite their differences in length and number, flagella and cilia share a common structural arrangement of microtubules called a “9 + 2 array.” This is an appropriate name because a single flagellum or cilium is made of a ring of nine microtubule doublets, surrounding a single microtubule doublet (axoneme) in the center (figure 18.6).
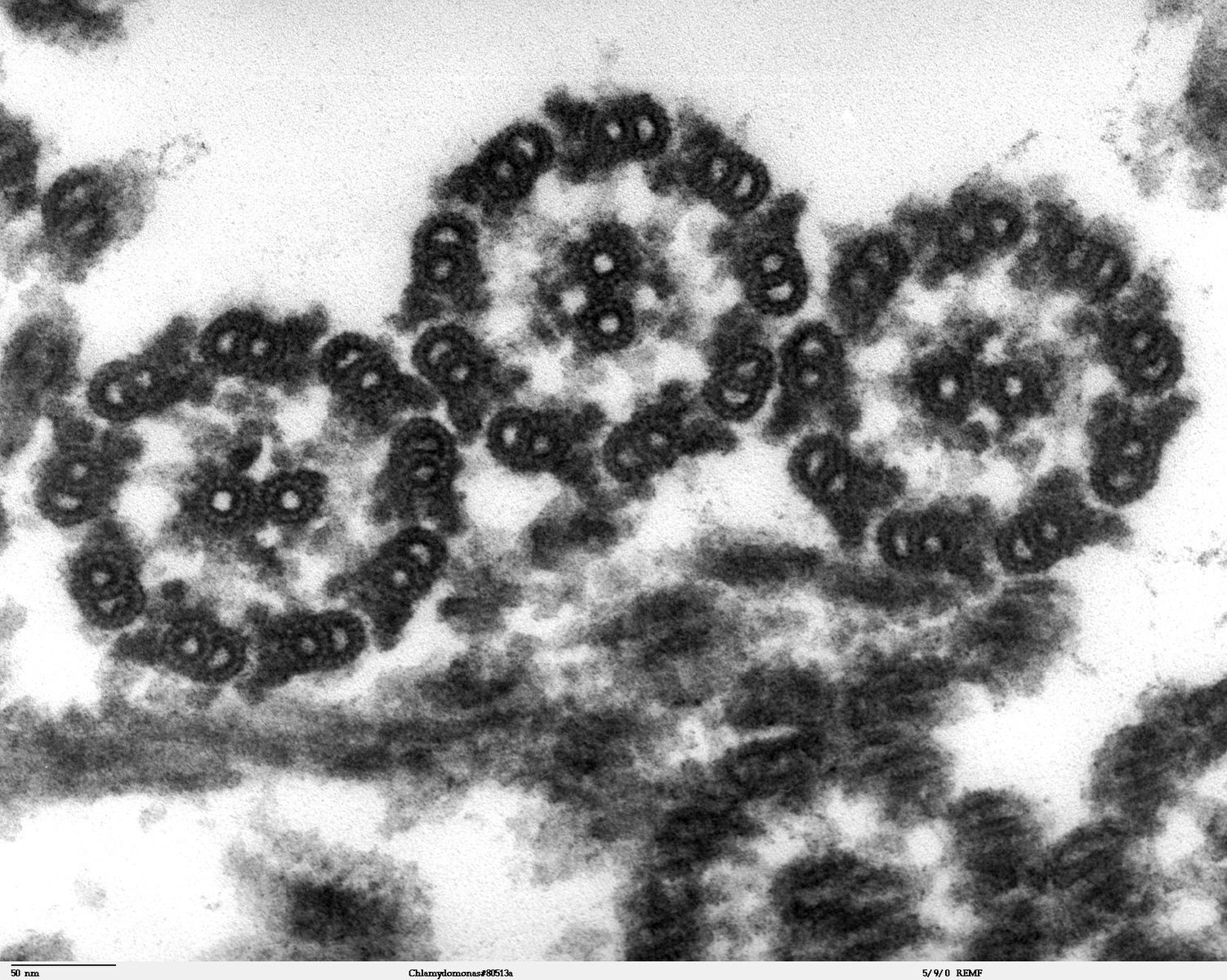
18.1 References and resources
Text
Clark, M. A. Biology, 2nd ed. Houston, TX: OpenStax College, Rice University, 2018, Chapter 4: Cell Structure, Chapter 5: Structure and Function of the Plasma Membranes.
Karp, G., and J. G. Patton. Cell and Molecular Biology: Concepts and Experiments, 7th ed. Hoboken, NJ: John Wiley, 2013, Chapter 9: The Cytoskeleton and Cell Mobility.
Le, T., and V. Bhushan. First Aid for the USMLE Step 1, 29th ed. New York: McGraw Hill Education, 2018, 48–49.
Figures
Dartmouth Electron Microscope Facility, Dartmouth College. Figure 18.6 This transmission electron micrograph of two flagella shows the microtubules’ 9 + 2 array: nine microtubule doublets surround a single microtubule doublet. Scale bar data from Matt Russell. Public domain. From Wikimedia Commons.
Grey, Kindred, Figure 18.1 Summary of the three major types of structural filaments. 2021. https://archive.org/details/18.1_20210926. CC BY 4.0. Adapted from Figure 3.18. CC BY 4.0. From OpenStax.
Grey, Kindred, Figure 18.2 Spatial organization of the three types of fibers… 2021. https://archive.org/details/18.2_20210926. CC BY 4.0. Adapted from Figure 4.22. CC BY 4.0. From OpenStax.
Grey, Kindred, Figure 18.3 Microfilaments are comprised of two globular protein intertwined strands, which we call actin. For this reason, we also call microfilaments actin filaments. 2021. https://archive.org/details/18.3_20210926. CC BY 4.0. Adapted from Figure 4.23. CC BY 4.0. From OpenStax.
Grey, Kindred, Figure 18.4 Several strands of fibrous proteins that are wound together comprise intermediate filaments. 2021. https://archive.org/details/18.4_20210926. CC BY 4.0. Adapted from Figure 4.24. CC BY 4.0. From OpenStax.
Grey, Kindred, Figure 18.5 Microtubules are hollow. Their walls consist of 13 polymerized dimers of \(\alpha\)-tubulin and \(\beta\)-tubulin. The left image shows the tube’s molecular structure. 2021. https://archive.org/details/18.5_20210926. CC BY-SA 4.0. Adapted from Microtubule structure esp by Posible2006. CC BY-SA 4.0. From Wikimedia Commons.
18.2 Cell Movement
Motor proteins, such as myosins, dyneins, and kinesins (figure 18.7), move along cytoskeletal filaments via a force-dependent mechanism that is driven by the hydrolysis of ATP molecules. Motor proteins propel themselves along the cytoskeleton using a mechanochemical cycle of filament binding, conformational change, filament release, conformation reversal, and filament rebinding. In most cases, the conformational change(s) on the motor protein prevents subsequent nucleotide binding or hydrolysis until the prior round of hydrolysis and release is complete.
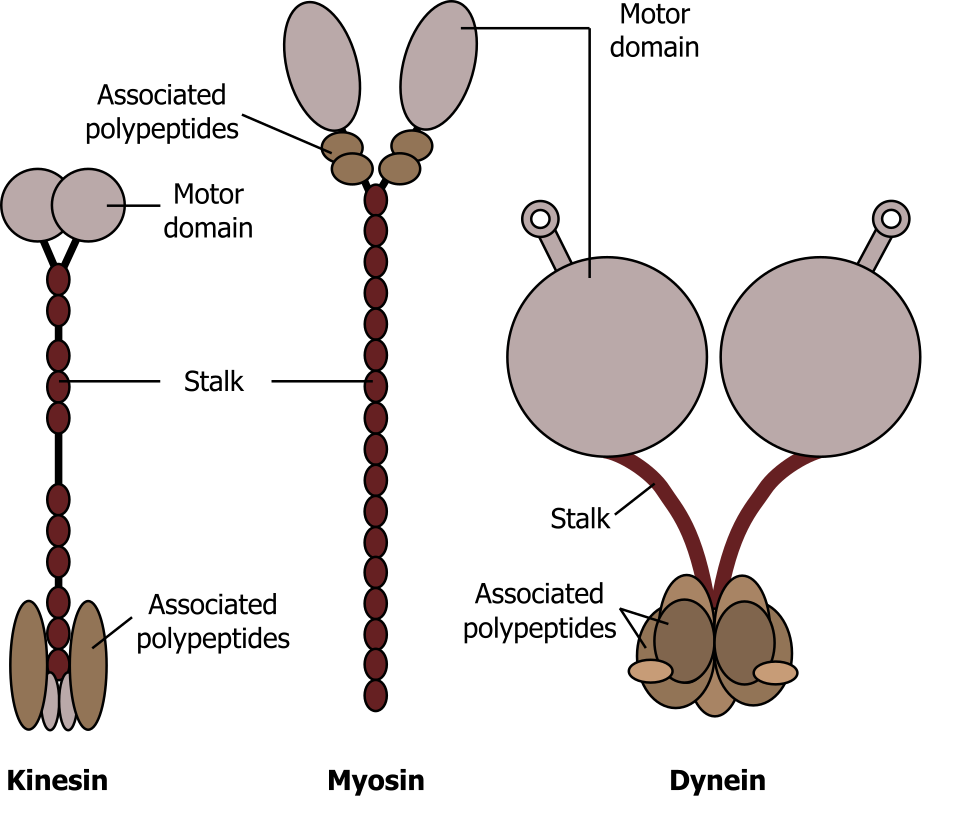
Myosin
Myosin can be characterized as conventional or unconventional, with characteristic head groups (that bind ATP) and unique tails. Myosin is essential for muscle contraction, and this occurs in striated muscle (skeletal and cardiac) after specific binding sites on the actin have been exposed in response to the interaction between calcium ions (Ca2+) and proteins (troponin and tropomyosin) that “shield” the actin-binding sites. Ca2+ is also required for the contraction of smooth muscle, although its role is different: here Ca2+ activates enzymes, which in turn activate myosin heads. All muscles require adenosine triphosphate (ATP) to continue the process of contracting, and they all relax when the Ca2+ is removed and the actin-binding sites are re-shielded.
Dynein
Dynein is a large motor protein that typically transports organelles (lysosomes or endosomes). It moves toward the minus end (α-tubulin) of microtubules, which is in the direction of the cell body.
Kinesin
Kinesin is a relatively small motor protein that moves membrane-bound cargo (e.g., vesicles). In contrast to dynein, most move toward the plus end β-tubulin) of the microtubules, which is typically away from the cell body. Figure 18.8 nicely summarizes the location and general role of each of these motor proteins.
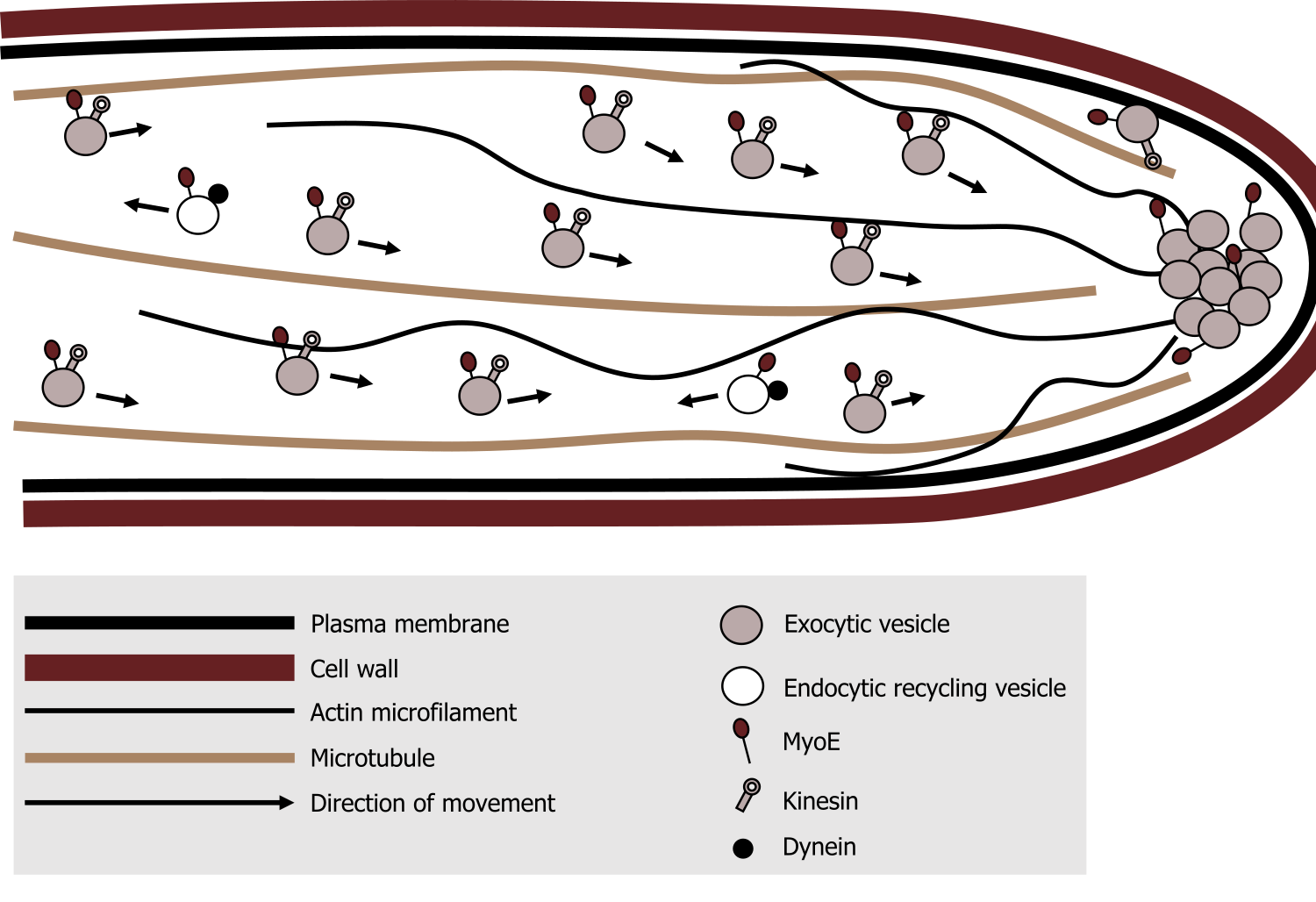
- Myosin can be found associated actin filaments, generally moving cargo (exocytic vesicles) away from the cell body. There is also a role in actin polymerization, which is essential for cellular motility.
- Kinesin is associated with microtubules moving cargo away from the cell body.
- In contrast, dynein, is associated with microtubules moving cargo (endocytic vesicles) toward the cell body.
18.2 References and resources
Text
Clark, M. A. Biology, 2nd ed. Houston, TX: OpenStax College, Rice University, 2018, Chapter 4: Cell Structure, Chapter 5: Structure and Function of the Plasma Membranes.
Karp, G., and J. G. Patton. Cell and Molecular Biology: Concepts and Experiments, 7th ed. Hoboken, NJ: John Wiley, 2013, Chapter 9: The Cytoskeleton and Cell Mobility.
Le, T., and V. Bhushan. First Aid for the USMLE Step 1, 29th ed. New York: McGraw Hill Education, 2018, 48–49.
Figures
Grey, Kindred, Figure 18.7 Comparison of the three different motor proteins. 2021. CC BY SA 4.0. Adapted from Aufbau der Motorproteine by keine Autoren genannt. CC BY SA 4.0. From Wikimedia Commons.
Grey, Kindred, Figure 18.8 Summary of the roles and movement of the motor proteins along various cytoskeletal elements. 2021. CC BY 4.0. Adapted from A simplified model for myosin V (MyoE) function at the hyphal tip in Aspergillus nidulans – journal.pone.0031218.g009B by Taheri-Talesh N, Xiong Y, Oakley BR. CC BY 2.5. From Wikimedia Commons.