9 Pleural Diseases
Learning objectives
- Distinguish the pathogenesis, pathophysiology, and clinical manifestations of pleural effusion, pleurisy, and pneumothorax.
- Relate the pathophysiology of pleural effusion and pneumothorax to lung and cardiac function.
While there are a few primary disorders of the pleura, their anatomy and location can make them susceptible to secondary diseases. The pleura fundamentally form a subatmospheric cavity within the thorax, making them susceptible to disorders that result in fluid accumulation (for example, cardiac failure). Conversely, the proximity of the pleura to other organs can mean pleural disease can affect other organs such as mediastinal structures and subdiaphragmatic viscera.
We will look at the most important and frequently occurring disorders of the pleura, namely:
- pleurisy,
- pleural effusion,
- pleural empyema, and
- pneumothorax.
To get warmed up, let us start with the simplest one, pleurisy.
Pleurisy
Pleurisy is synonymous with pleuritis and refers to inflammation of the pleural membranes that may be accompanied by pleural exudate or may remain “dry.” Inflammation of the membranes may be mild and transient due to common conditions such as bacterial or viral infection, or it may be severe and chronic and indicative of more serious conditions, such as lupus and rheumatoid arthritis.
The inflammation causes thickening of the membranes that may cause them to impinge on the pleural space. Prolonged inflammation leads to an accumulation of pleural macrophages, and these in turn initiate a proliferation of fibrocytes. The inflammatory process may prevent the membranes from moving freely, and in some patients the two inflamed membranes sliding against each other can produce an audible pleural friction rub, which sounds like leather rubbing against leather or walking on fresh snow. The hallmark symptom of a pleural rub is sudden onset chest pain associated with inhalation and cough.
In prolonged pathological conditions, the membranes may adhere, and calcium deposits can appear in old pleural fibrosis and are often associated with chronic conditions such as asbestosis. When significant, this level of fibrosis may restrict respiratory movement.
Pleural Effusion
In our description of pleurisy we eluded to our next pleural disorder, pleural effusion. The normally small amount of pleural fluid is sufficient to lubricate the movement of the lungs and is not detectable on x-ray without specialized radiography. Pleural effusion is the abnormal accumulation of fluid in the pleural space and is easily detectable. As with the formation of fluid accumulation in any part of the body, a normal fluid level in the pleural space is dependent on a balanced rate of formation and reabsorption. In pleural effusion, this balance is lost.
Initially it was thought that pleural fluid was formed by hydrostatic pressure in capillaries in the parietal membrane pushing fluid into the pleural space, and the fluid was then absorbed by capillaries in the visceral membrane. However, there is mounting evidence to show that reabsorption is actually performed by the lymph vessels in the parietal membrane. The low hydrostatic pressure and large capacity of these vessels helps maintain the normal, small volume of pleural fluid. Pleural effusion can be caused by:
- too much fluid formation,
- too little fluid absorption, or
- a combination of both.
For example, pleural effusion in cardiac pulmonary edema occurs as fluid leaks across the visceral pleura from the lung.
Two broad classifications exist, transudate and exudate, so let us compare them now (summary in table 9.1).
Transudative effusion occurs when there is a disturbance in the Starling’s forces influencing fluid movement across the capillary. As such transudate can be caused by an increase in hydrostatic force pushing fluid out of the capillary, such as in congestive heart failure, or a decrease in the plasma oncotic pressure retaining fluid in the capillary, such as in kidney or liver disease. This results in a transudate with low specific gravity, protein concentration, and cell count.
Exudative effusion is caused by increased capillary permeability, such as that caused by the inflammatory process. Relate this back to your understanding of exudative pleurisy (figure 9.1). As larger molecules can exit the leaky capillaries, exudative effusion has a higher specific gravity, higher protein concentration, and likely a higher cell count.
Transudate | Exudate |
---|---|
Increased hydrostatic pressure | Increased capillary permeability |
Decreased plasma oncotic pressure | |
- Low specific gravity - Low protein concentration - Low cell count |
- Higher specific gravity - Higher protein concentration - Increased cell count |
Table 9.1: Comparison of transudative and exudative pleural effusions.
The manifestation of pleural effusion varies with the quantity of fluid accumulation and the time line. Symptoms may in fact be absent when the effusion is small. In the case of exudative pleurisy, an initial rub pain may disappear as exudate accumulates and separates the rubbing pleural surfaces.
As the effusion volume increases the patient is likely to experience dyspnea and physical exam will more likely include dullness to percussion and absence of breath sounds as the effusion forms a fluid pillow around the lung.
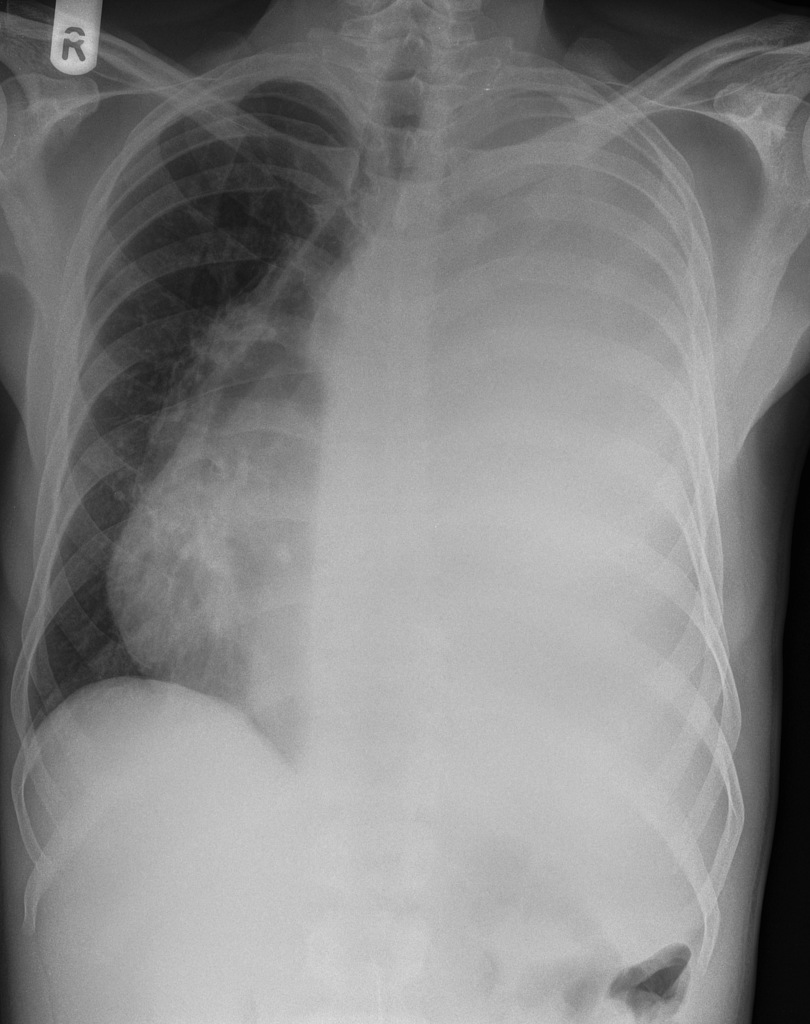
As the severity of the effusion increases the patient will be severely short of breath, and the risk of the effusion pushing mediastinal structures to the contralateral side becomes significant and urgent (figure 9.1) as even mild displacement of the mediastinum can reduce cardiac output and produce hypotension. More significant displacement can become life threatening.
As we have already mentioned, the pleural fluid’s composition can provide a great deal of information about the underlying mechanism. We have already mentioned the ability to distinguish between an exudate and a transudate by analysis of the fluid. But gross inspection of the fluid can be revealing before lab tests are ready.
Fluid drawn through thoracentesis that appears clear and straw colored (figure 9.2) is likely to be transudate. The clarity of the fluid relates to a low protein content reflecting intact capillaries, and you should be looking for disturbances in Starling’s forces. Lab results can confirm the integrity of the capillary beds. Clinically the pleural fluid’s protein content and lactate dehydrogenase concentration are used and are expressed as a ratio with the patient’s plasma concentrations. Light’s criteria set diagnostic thresholds for these ratios to determine transudative or exudative effusions.
The higher protein of exudative effusion gives the fluid a more turbid or cloudy appearance, and high protein content may even give the fluid a foamy head (figure 9.2). Further lab analysis of the composition and cell content of the exudate can help determine the underlying cause of the leak. For example, presence of polymorphonuclear leukocytes is highly suggestive of pyrogenic infection, whereas predominance of lymphocytes is indicative of TB or malignancy.
Presence of lysed red blood cells will give the fluid a red turbid appearance (figure 9.2) and is indicative of trauma or malignancy, and again will require further investigation. If a malignancy has penetrated the lymphatics, such as the thoracic duct, the resultant chylous exudate will have a milky appearance.
A purulent fluid (figure 9.2) is indicative of infection within the pleural space and culturing the fluid will allow the pathogen to be determined. When pleural fluid is grossly purulent or contains pyogenic organisms we refer to this as pleural empyema. The most common route of entry for organisms is from underlying pneumonia or lung abscess, or through penetrative surgery or chest wound. The patient usually presents with fever and other manifestations of bacterial infection.
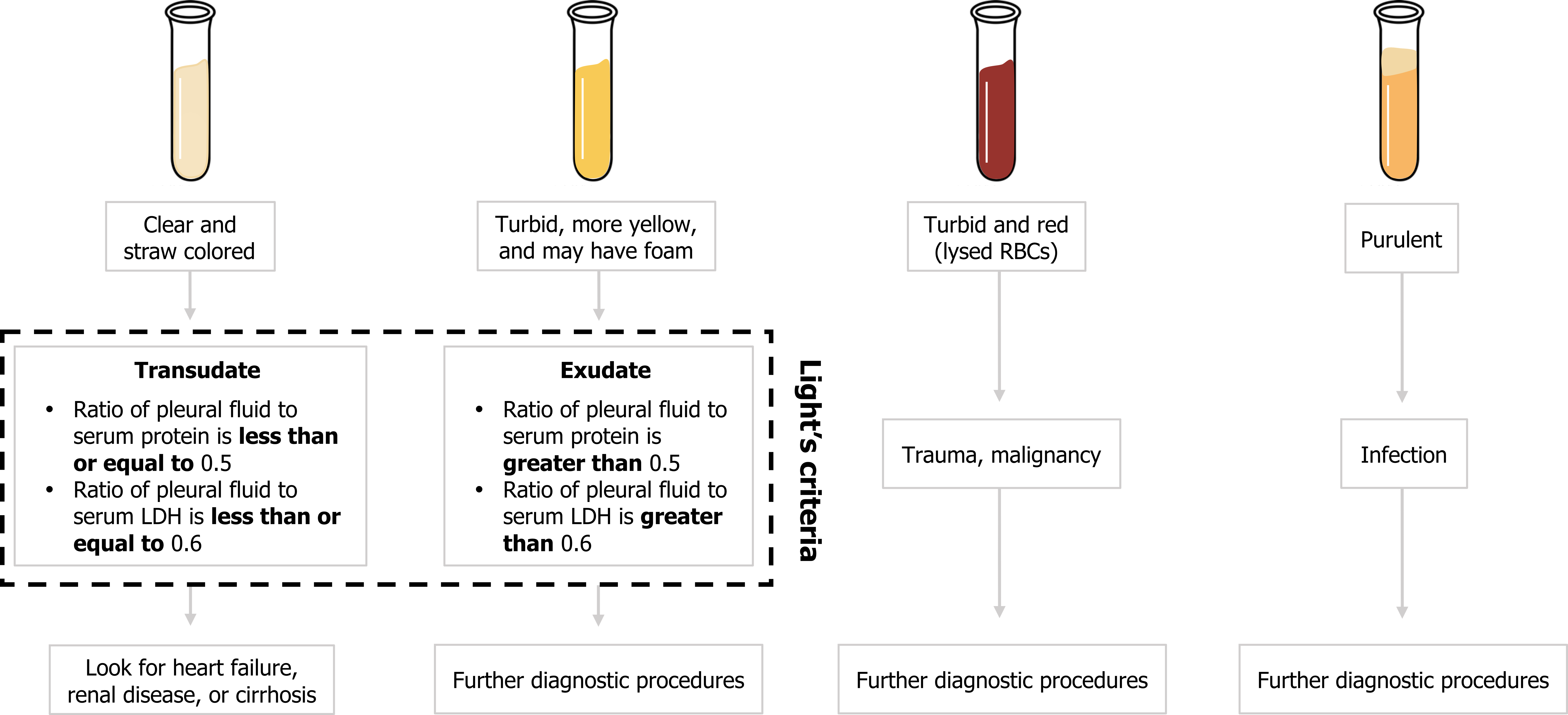
Pneumothorax
Normally the negative pressure inside the pleural space opposes the lung’s recoil and holds the lung surface to the interior of the thorax. If the pleural membranes are disrupted this subatmospheric or negative pressure is lost and the lung can recoil, or collapse. This is a pneumothorax.
The causal events of losing the negative pleural pressure divide pneumothoraces into one of two categories: spontaneous or traumatic. (A third category exists, artificial pneumothorax, which was intentionally induced as part of procedures that are very rarely used today.)
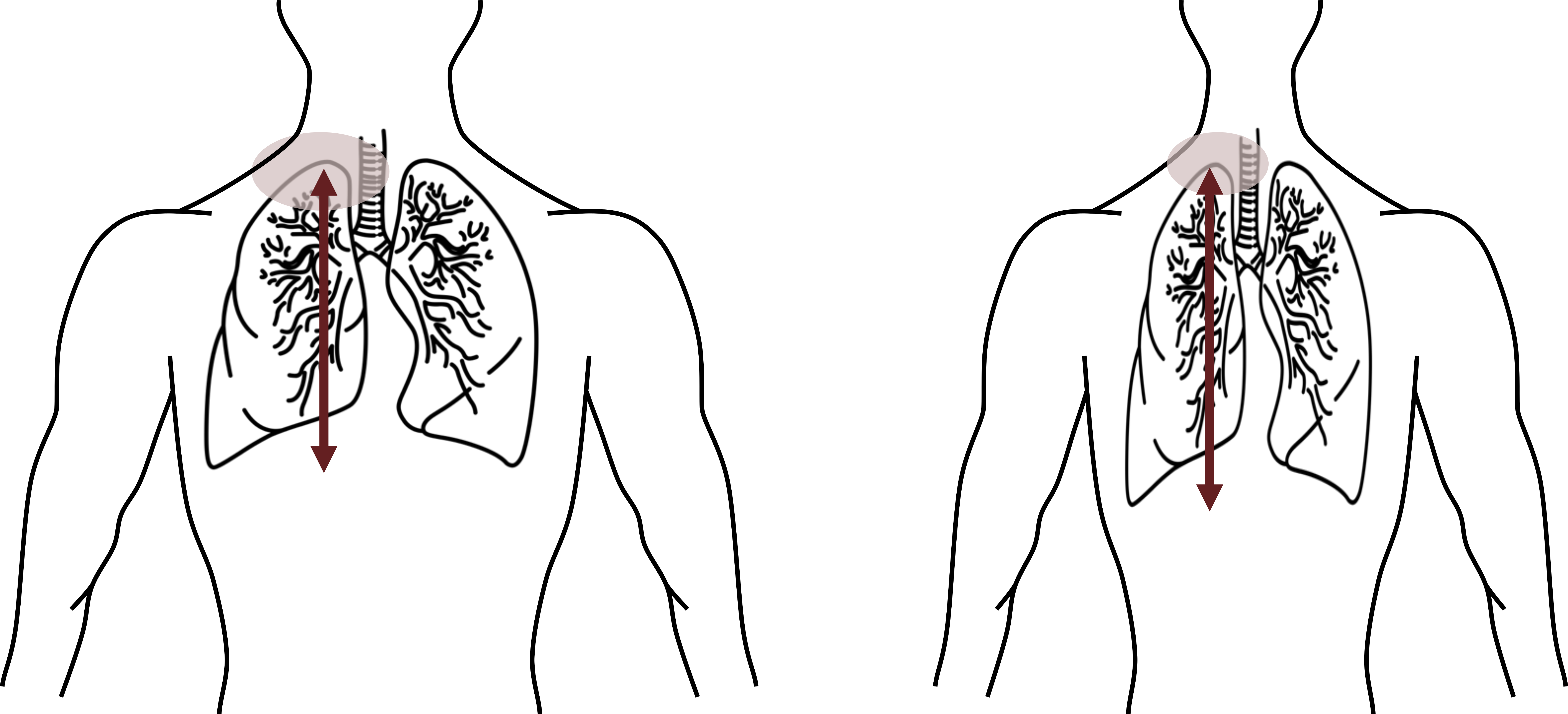
Spontaneous pneumothorax occurs in the absence of accidental or intentional trauma. When it occurs in otherwise healthy individuals it is usually the fault of anatomy and occurs mostly in tall, thin individuals with a long and narrow chest. If you compare the two body morphs in figure 9.3, the tall, thin torso has a lot of lung mass hanging below a relatively small apical section of pleural membrane compared to the other torso.
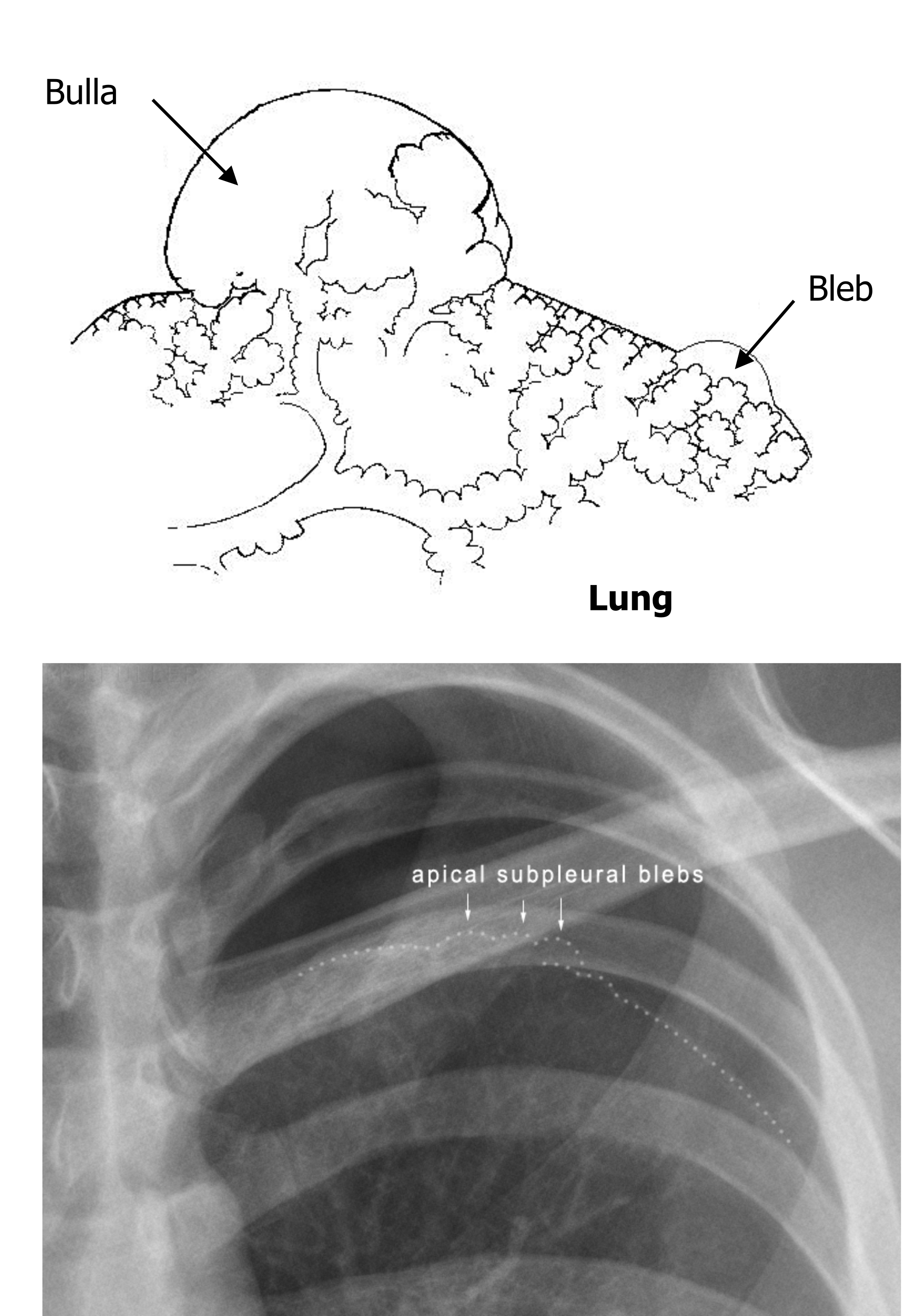
The proportionately greater weight of lung per unit surface area at the apex increases the risk that bulla form (figure 9.4), then rupture causing loss of pleural membrane adhesion.
The most common cause of spontaneous pneumothorax in pulmonary patients is a ruptured bleb or bulla. These thin-walled, air-filled cavities are found near the pleural surface, particularly in cases of emphysema. In the past, TB was thought to be the most common cause of pneumothorax as its destructive path encroached on the pleural space.
Traumatic pneumothorax is often a consequence of chest injury that involves laceration of the pleura. This might be due to a broken rib or penetrative wound to the chest that allows air to enter the pleural space and equilibrate with the atmosphere. The injury might not be malicious though as some procedures might inadvertently or unavoidably perforate the pleura, such as thoracentesis, pleural biopsy, lung biopsy, or subclavian vein puncture. Trauma from within the lung may also occur. Similar to the mechanism of a rupturing bleb, if excessive airway pressures are used during mechanical ventilation there is the risk that airspaces are disrupted and barotrauma involves the visceral pleura.
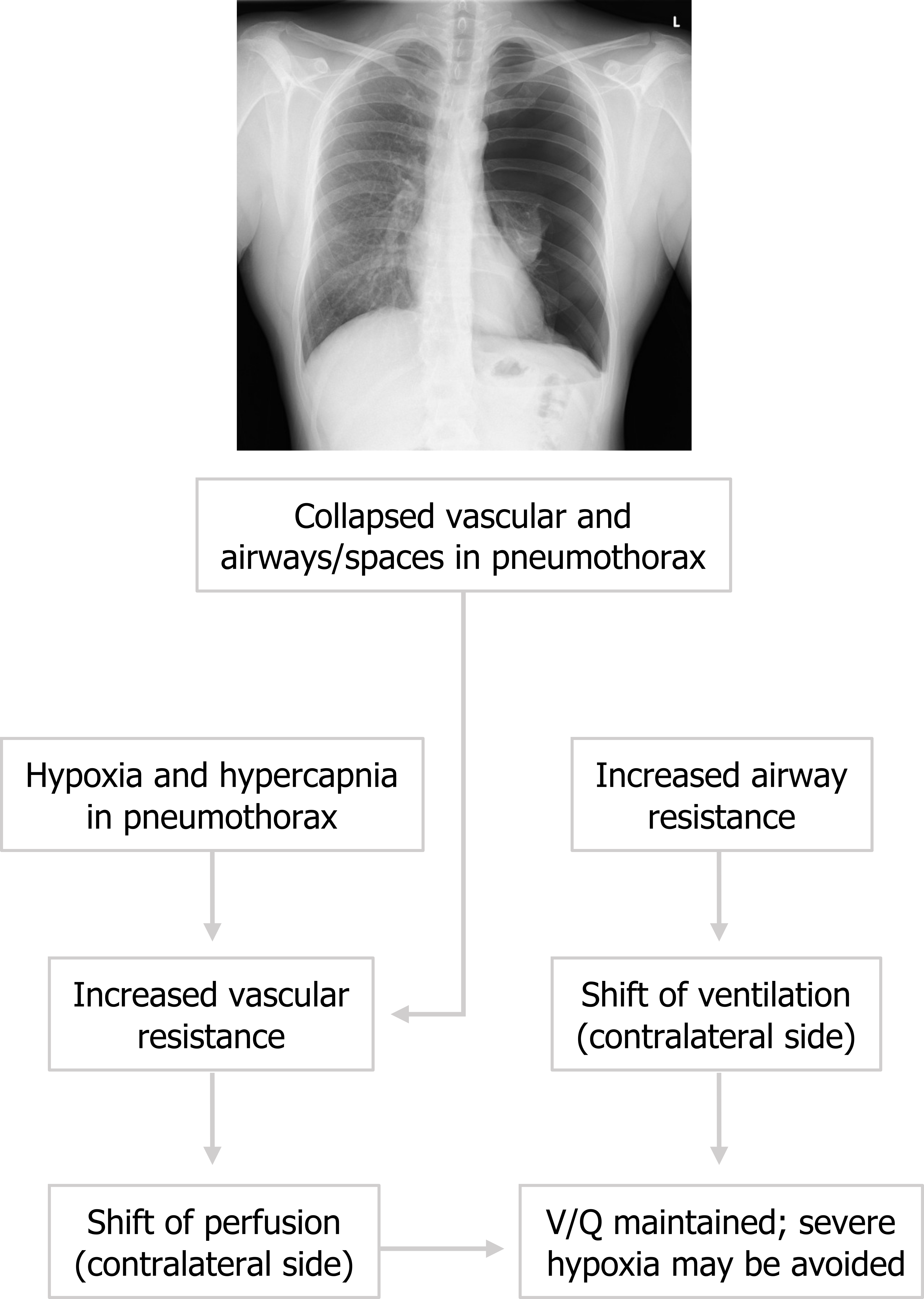
The pneumothorax is readily identified with translucency on the affected side. The pathophysiology of a pneumothorax (figure 9.5) leads to hypoxia and hypercapnia in the affected lung through changes in perfusion and ventilation. Perfusion to the affected side is markedly reduced as the vasoconstrictive response to local hypoxia and the loss of radial traction to vessels profoundly increases vascular resistance. Consequently, perfusion shifts to the contralateral and unaffected lung. Similarly ventilation to the collapsed lung is minimal as airspaces are collapsed and airway resistance is very high, but the chemoreceptive reflexes increase ventilation to the unaffected lung where resistance is normal. Because perfusion and ventilation are shifted to the normal lung, V/Q mismatching may be compensated to some degree and severe hypoxemia may be avoided. When pneumothorax occurs patients usually describe a rapid onset of dyspnea and initial sudden sharp pain. The pain often then transitions into a dull ache.
Tension pneumothorax may arise if the disruption to the pleural is such that the injury acts like a valve. During inspiration when thoracic pressure falls air enters the airways and also the thoracic cavity via the injury. During expiration when the thoracic pressure increases, the wound closes, stopping the air that entered the thoracic cavity from leaving. So with each inspiration more air enters while little or none leaves during the subsequent expiration. The accumulating volume can begin to push the heart and mediastinum to the contralateral side. This may severely affect cardiac output and rapidly become life threatening.
These CT scans in figure 9.6 shows a spontaneous pneumothorax (left panel) and a tension pneumothorax (right panel) with significant shift of the mediastinum over the contralateral hemithorax. So now you should be able to distinguish between the causes and mechanisms of these pleural disorders and determine their effects on pulmonary and cardiac function.
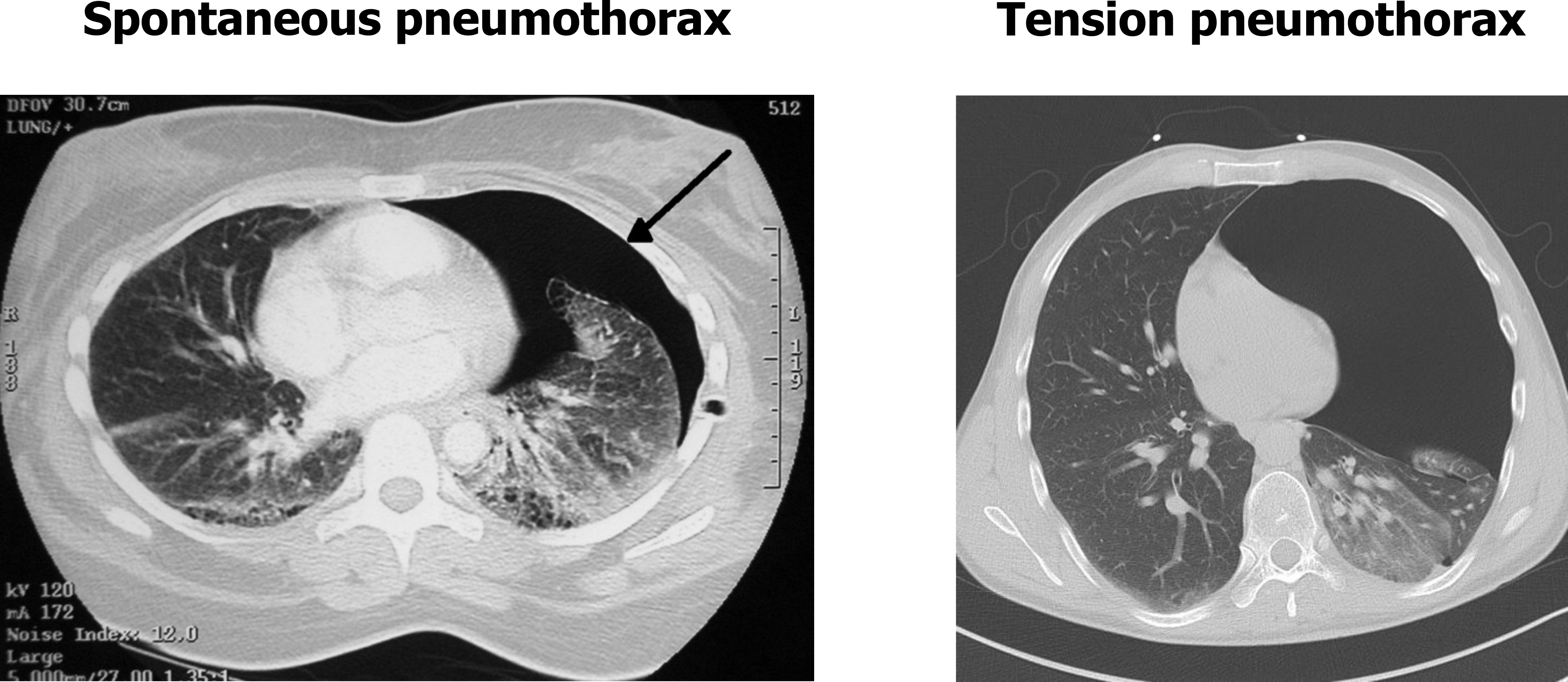
Farzan, Sattar, with Doris L. Hunsinger and Mary L. Phillips. “Chapter 22.” In A Concise Handbook of Respiratory Diseases. Reston, VA: Reston Publishing Company, 1978.
Husain, Aliya N. “Chapter 15: The Lung.” In Robbins and Cotran Pathologic Basis of Disease, 9th ed., edited by Vinay Kumar, Abul K. Abbas, and John C. Aster. Philadelphia: Saunders, an imprint of Elsevier Inc., 2015.
West, John B. “Chapter 5: Restrictive Diseases.” In Pulmonary Pathophysiology: The Essentials, 7th ed. Baltimore: Lippincott Williams & Wilkins, a Wolters Kluwer business, 2008.
Figures
Figure 9.1: Severe pleural effusion leading to mild displacement of the mediastinum. Bickle, I., et al. 2017. CC BY-NC-SA 3.0. From https://doi.org/10.53347/rID-56506.
Figure 9.2: Appearance of pleural fluid and diagnosis. Grey, Kindred. 2022. CC BY 4.0. Added Test Tube by Victoria Codes from Noun Project (added color)(CC BY 3.0). https://archive.org/details/9.3_20220203
Figure 9.3: A tall thin body morph (right) is more prone to a spontaneous pneumothorax. Grey, Kindred. 2022. CC BY 4.0. Added Lungs by iconmu from Noun Project (CC BY 3.0). https://archive.org/details/9.4_20220203
Figure 9.4: Rupturing bulla and blebs can lead to spontaneous pneumothorax. Grey, Kindred. 2022. Includes Pneumot rax bullae by Robertolyra from WikimediaCommons (public domain) and JZTg XeWvwrS45Dzl7SoSg288930 by Travis from Wikiradiography/MediaWiki (CC BY-SA 3.0). https://archive.org/details/9.5-new
Figure 9.5: Pathophysiology of a pneumothorax. Grey, Kindred. 2022. CC BY-NC-SA 3.0. Added case 1 by Gorrochategui, M., Niknejad, M., et al. from https://doi.org/10.53347/rID-4578 (CC BY-NC-SA 3.0). https://archive.org/details/9.6-real
Figure 9.6: CT comparison of an uncomplicated pneumothorax and a tension pneumothorax. Grey, Kindred. 2022. CC BY-NC-SA 3.0. Includes Pneumothorax CT by Doc James from WikimediaCommons (CC BY-SA 2.5) and image 1 by Wichmann, J., et al. from https://doi.org/10.53347/rID-25861 (CC BY-NC-SA 3.0). https://archive.org/details/9.6_20220203