Chapter 6: Soil Properties and Management
“The dirt on Virginia soil”
“To fertilize or not to fertilize, that is the question.” (With apologies to Shakespeare)
Chapter Contents:
Introduction
Our Virginia soils differ based on soil forming factors and physiographical location. Geological impacts of the tectonic plate interactions, weathering, climate, hydrology, ocean level, and human utilization of the land has changed the particle sizes, mineral content, and microflora of soils across the state. This chapter provides an overview of the formation of natural Virginia soils, nutrient content, and characteristics of the soils, their geographical distribution, and the effects of human interventions: farming, silviculture, and urban/city development on the soil.
Due to previous land utilization or urban/city development activities, soils often need to be amended to adjust the pH, balance the nutrients, reduce soil compaction and design drainage issues in order to promote vigorous disease-free tree growth.
Learning Objectives
Upon completing this chapter, the student should be able to:
- Identify physiographical provinces of Virginia,
- Identify soil types, and their properties
- Determine the soil type, mechanical manipulations, and nutrient amendments that will be required to support successful tree growth in a natural or urban/city (landscaped or managed) site based data obtained by soil analysis.
Review: VCE Master Gardener Handbook 2015 (9/18 update)
- Chapter 3 Soils and Chapter 4 Nutrient Management and Fertilizers.
Soil Formation and Properties
Soil properties are the effects of physical, chemical, and biological processes determined by the parent material, climate, topography, organisms, time, and human intervention.
Physical Properties
Texture indicates the relative content of various sizes of particles in the soil. Sand is 0.05 mm – 2mm (Made of small rock fragments from the size that fits inside of your fingerprint grooves to less than about 1/8 inch.), silt is 0.002 mm – .05 mm (even smaller rock fragment particles), and clay is <0.002 mm (a microscopic secondary mineral that has complex structure and is very attractive to water and nutrients and other ions in the soil. Iron oxides are clays as well.). Texture influences the ease with which soil can be worked, the amount of water and air it holds, and the rate at which water can enter and move through soil. Texture is considered to be a permanent property. Total surface area increases as particle size decreases. Clays have higher surface area than sands.
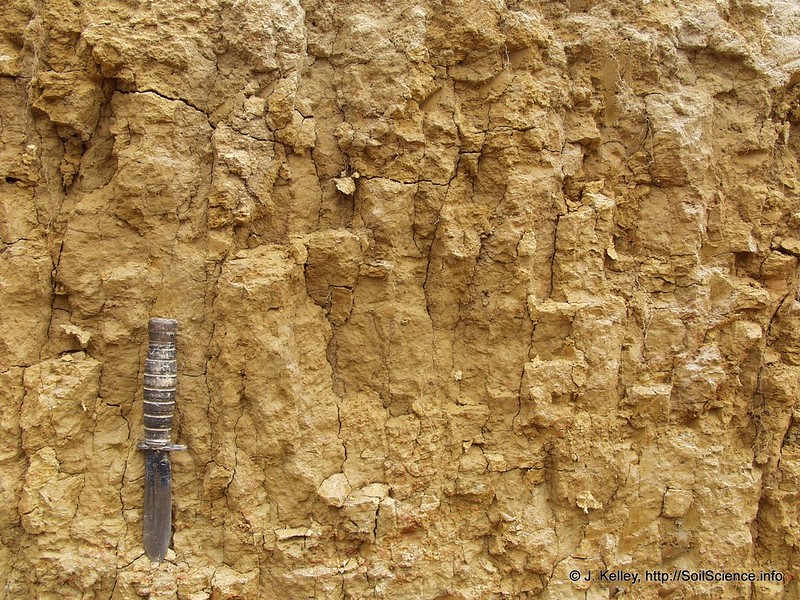
Structure refers to the arrangement of soil particles into units called soil aggregates. An aggregate possesses solids, pore space, microbes, animal organisms, and sometimes live roots. Soil structure can be permanently damaged or destroyed by plowing or compaction but can also be restored with rough physical processes such as freeze-thaw or wet-dry cycles or through the addition of organic matter and the activity of organisms that increase pore space.
Texture + structure influence = soil behavior
Organic Matter consists of plant and animal residues at various stages of decomposition, cells and tissues of soil organisms, and substances synthesized and exuded by soil organisms. Stable, highly decomposed organic matter is called humus.
Compaction is the process in which a stress applied to a soil causes loss of pore space, such as equipment traffic on wet soils. The measure of soil bulk density, which is the ratio of dry weight of a soil sample divided by its volume, determines the bulk density or compaction. As the dry weight increases relative to the volume, porosity in the soil decreases and compaction increases. This situation restricts root, air and water movement and leads to plant stress. Urban soils tend to be moderately to heavily compacted because of the amount of traffic they receive during construction or landscaping.
Drainage removes excess free water from the soil. Water-filled soil pores may become depleted of oxygen over time and the water prevents air and oxygen from getting to plant roots from the atmosphere.
Color is mainly produced by the minerals (or lack thereof) and water present and by the organic matter content. In general, darker colors indicate higher humus content; redder colors indicate higher iron oxide content and good drainage; and gray colors indicate long-term water saturation.
Odor of productive soils (aerobic) should smell fresh, clean and pleasant or have little odor at all. If the soil smells like ammonia or has a rotten egg odor that is a good indication there is poor drainage or lack of oxygen in the soil (anaerobic).
Temperature is the measurement of the warmth in the soil. Ideal soil temperatures for most plants are 65 to 75 F.
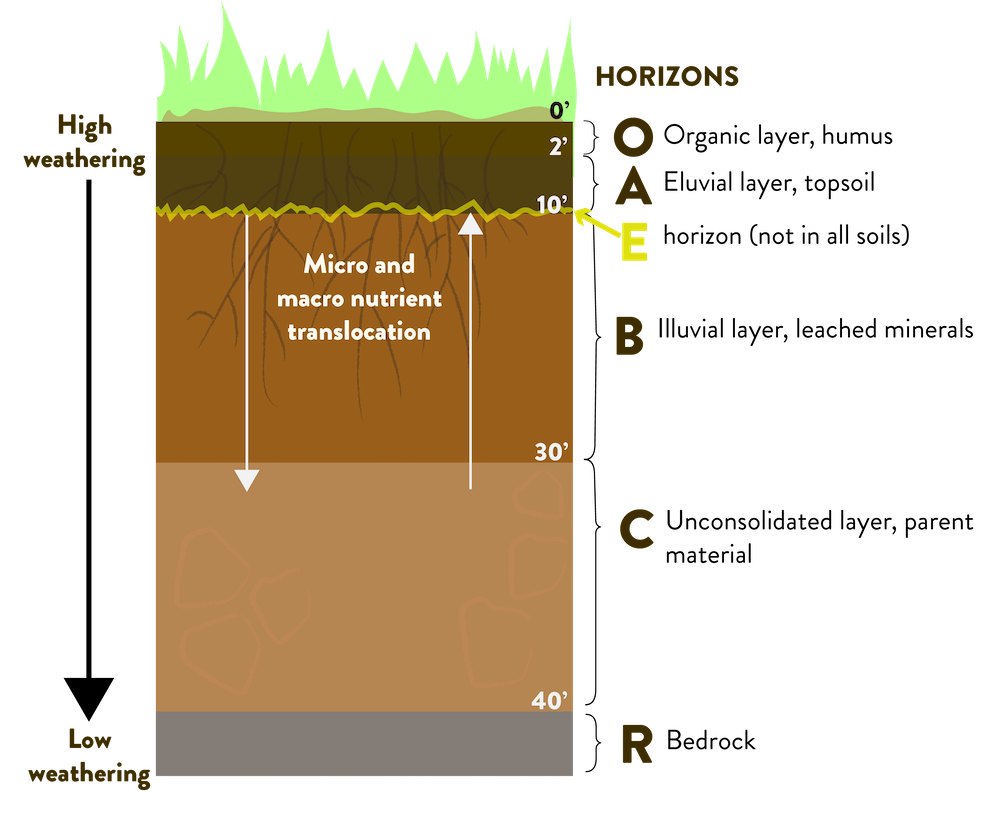
All tree roots require three soil elements: water, oxygen, and soil compaction levels low enough (or with void spaces sufficiently large enough) to allow root penetration. If all these conditions are met, and the tree has the genetic potential, roots can grow to great depths. Under ideal soil and moisture conditions, roots have been observed to grow to more than 20 feet (6 meters) deep.[1] In many urban/city planting sites ideal root-growing conditions often require restoration efforts.
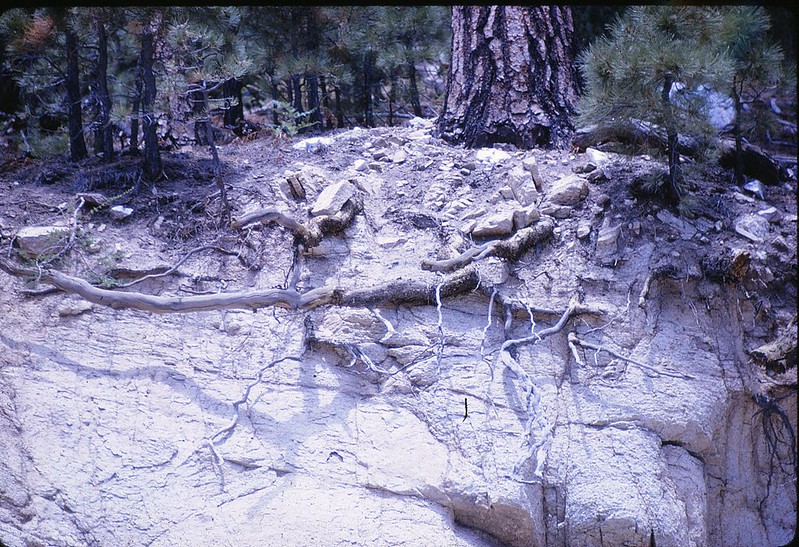
In most soil profiles, the largest numbers of roots are found in the ‘A’ and ‘B’ Horizons.
Chemical Properties
There are 17 known essential elements for plant nutrition. In relatively large amounts, the soil supplies nitrogen, phosphorus, potassium, calcium, magnesium, and sulfur. The majority of these elements are taken up by plants in ionic form, many of which are cations which have positive charges. Nutritional cations include potassium (K+), calcium (Ca++), magnesium (Mg++), and ammonium (NH4+). Other cations include hydrogen (H+) and sodium (Na+). Aluminum (Al3+) is also a potentially toxic cation that is more readily taken up as pH becomes more acidic. Phosphorus forms many net negatively charged compounds called anions. An example is P2O5-2, a dehydrant.
Cation Exchange Capacity (CEC)
The Cation Exchange Capacity (CEC) is a measure of the net negative charge per unit of clay. Nutrient cations are readily attracted to clay particles which have a net negative charge. A water molecule’s positive polar ends are also attracted to clay. Humus also has a high CEC and may also be adsorbed to clay. If the CEC is too low, cations and water are not adsorbed strongly and are easily drained or leached away. Soils with little clay, such as sand textures, and highly weathered clays and oxides have very low CEC. The CEC determines a soil’s ability to adsorb nutrients, and is related to potential fertility. Although many factors affect cation exchange between the clays or humus and the plants, we are most interested when nutrient cations and anions are exchanged across root cell walls and taken into the root. The plant root releases hydrogen ions, which then replaces the cations in the soil for uptake into the plant. Uptake of phosphate anions requires some energy expenditure by the plant.
pH is a measure of the acidity and alkalinity in soils on a scale of 1 to 14. The optimal pH range for most trees is between 5.5 and 6.5.
Salinity is the salt content in the soil. Sulfates, carbonates, and chlorides are salts.
Heavy Metals are lead, chromium, arsenic, zinc, copper, cadmium, mercury and nickel. “They rarely cause problems with plants at the levels found in urban soils.”[2] Heavy metals such as zinc and copper are also micronutrients essential to plant health and development.
Biological Properties: Aerobic, Anaerobic, Fauna, Microflora
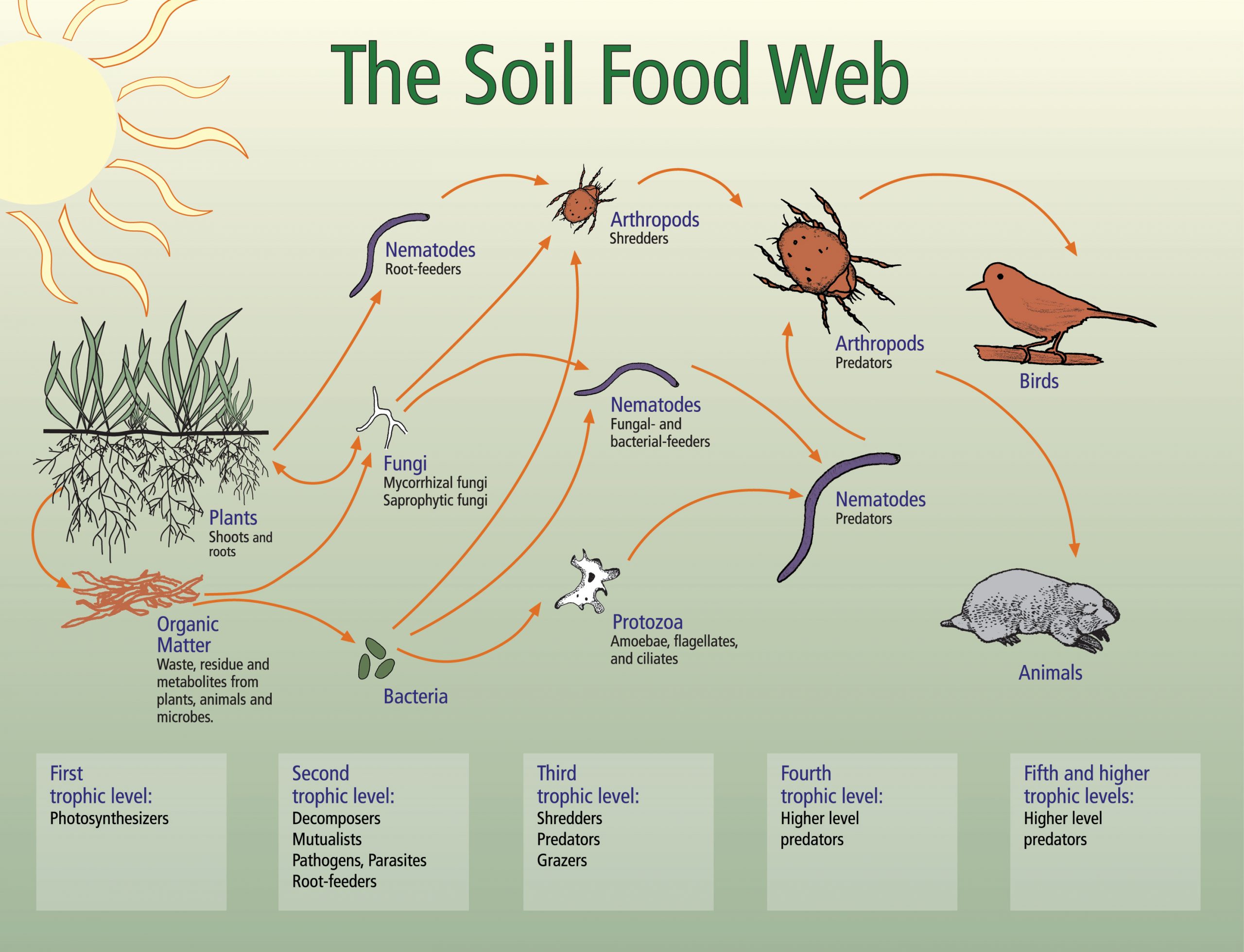
Microbial soil crusts play an important role in regulating nutrient cycling, biomass production, soil stability, and water infiltration. More in-depth information on the soil food web is available in the Master Gardener Training Manual.
Virginia Physiographical Provinces and Soil Orders
Numerous geological tectonic plate interactions and climate extending variations from 250 to 540 million years ago resulted in a great variation in geologic resources. Virginia has a very diverse physiographical landscape. Virginia is divided into five main physiographical provinces as a result. Soils have developed from a broad range of parent rock and vegetation materials. Soils are not static and are constantly being moved and changed by gravity, water, wind, and man. “Consequently, the numerous classified soil types that cover the state exhibit great variation in depth, textural and mineral composition, organic matter content, water-holding capacity, pH, fertility, and other characteristics. Drastically different soils may occur within the same landscape. Several soils may occur together within a field of a few acres.”[3] This is not necessarily the case with urban soils areas where soils have been variably changed by human interaction.
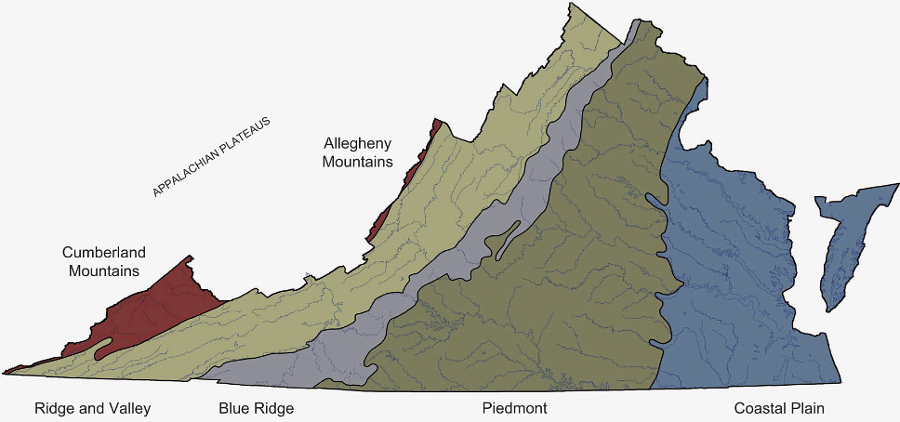
The Cumberland Mountains province (also known as the Allegheny Plateau) covers a portion of Southwest Virginia in Lee, Wise, Dickinson, and Buchanan Counties. Broad summits are deeply dissected by steep, narrow valleys. Level bedrock is dominantly sandstones under the summits and sandstone interbedded with siltstones, shale, and coal below. The area is largely forested with temperate broadleaf and mixed forests. Ultisols dominate on more gentle slopes while Inceptisols occur on steeper areas and along streams. Entisols occur in very gravelly active stream and river floodplains.
The Ridge and Valley province is a series of roughly parallel long narrow ridges and valleys along the western edge of the Commonwealth. The highest ridges are underlain by resistant sandstones and conglomerates, with shales on sideslopes, while the valleys are underlain by more easily weathered limestones, dolomites, and shales. Thick deposits of colluvium occur on mountain footslopes. The Shenandoah Valley is very fertile and has been dominated by agricultural land uses for centuries, while the steeper ridges have often remained in forestland[4]. Soils supporting mixed forest growth, Ultisols and Alfisols occur on gentle slopes and Inceptisols occur on shallow soils near rock outcrops, in and very steep areas and in some floodplains. Mollisols occur in some floodplains with significant limestone in the watershed.
The Blue Ridge province consists of a narrow, irregularly weathered series of peaks underlain by a core of resistant granites, gneisses, and greenstone, with resistant metasedimentary rocks (quartzite, metasiltstone, and phyllite) exposed on the western flank.[5] Much of the area is forested and species vary tremendously with elevation, aspect, soil, and disturbance history.[6] The highest elevations such as the Grayson Highlands support Spruce/Fir and the lower regions support temperate broadleaf and mixed forests. Soils found in these areas are usually Inceptisols on the higher peaks and Ultisols on lower slopes and in coves.
The Piedmont province comprises the central one-third of the Commonwealth. It is a rolling to hilly landscape that lies between the Blue Ridge on the west and the Coastal Plain on the east. Most of the province is covered by a thick mantle of soil with soft, highly decomposed former bedrock underneath, often rich in clay and mica. Deep weathering and leaching for millions of years caused loss of many of the original chemical constituents, notably base metals. The Triassic Basins (former rift valleys that occur scattered across the Piedmont) are filled with transported sediments that formed into shales, siltstones, sandstones and conglomerates.”[7] The area was extensively cleared and farmed until the Civil War. Many soils are badly eroded from that time. Forests are mixed hardwoods and evergreens, with many loblolly pine plantations. Soils are predominately Ultisols with some Inceptisols near streams. Alfisols and Ultisols occur in the Triassic basins.
The Coastal Plain province covers the eastern one-third of the Commonwealth east of the Piedmont and the Fall Line. The soils formed from transported materials eroded from the other provinces and deposited in rivers or in the ocean. Bedrock is buried very deeply. The terrain is gently sloping except along streams. The forests are mixed hardwoods and evergreens, with many loblolly pine plantations on uplands and swamps along most floodplains. Most older soils are Ultisols and younger soils are Alfisols, with some Histosols in marshes along estuaries, Entisols along ocean shorelines, and Spodosols along sandy river systems such as the Blackwater River.
(Note: For a listing of trees that grow naturally in the different physiographical regions of Virginia refer to: Virginia Department of Conservation and Recreation, Division of Natural Heritage. “Overview of the Physiography and Vegetation of Virginia”).[8]
Soil orders are based on 2 or more physical or chemical characteristics that differentiate them from one another. Soil surveys of the state’s naturally occurring soils indicate 7 of the 12 USDA, NRCS soil orders are prevalent throughout the state. These are in the order of occurrence in Virginia by percentage:
- Ultisols (last formed) are highly weathered and strongly leached, infertile mineral soils with significant subsoil clay accumulation that formed under deciduous, coniferous, or mixed forest and woodland vegetation. Farming, silvopasture, and silviculture requires inputs of lime and fertilizer.
- Alfisols are moderately leached soils with significant subsoil clay accumulation and relatively high natural fertility. These soils have mainly formed under forest and have a subsurface horizon in which clays have accumulated. The higher mineral content results in a more productive soil and permits a greater variety of crops than Ultisols.
- Inceptisols (beginning) are mineral soils of relatively new in origin and are characterized by having subsoil horizons just beginning to exhibit a moderate degree of soil development. Inceptisols lack significant clay accumulation in the subsoil and may be naturally fertile or infertile.
- Entisols (newly formed) exhibit little soil development other than the presence of an identifiable topsoil horizon. These soils occur in unstable environments of recently deposited sediments such as active flood plains, dunes, and landslide areas. They may be naturally fertile or infertile.
- Mollisols (soft, deep, fertile) are the soils forming in alluvium eroded from limestone and dolomite bedrock. They are characterized by a thick, dark surface horizon which results from the long-term addition of organic matter. Mollisols are extensively used for forests in Virginia but are among the most productive and fertile soils of the world. They occur in flood plains draining limestone and dolomite such as the Shenandoah, Roanoke, and James River.
- Spodosols (sandy, acidic) have a strongly leached surface layer and a subsoil in which an amorphous mixture of organic matter and aluminum, with or without iron, accumulates in a subsoil horizon. Most Spodosols have little silicate clay and have the appearance of white sugar sand. These are soils formed under coniferous forests such as the longleaf pine growing near Zuni.
- Histosols (organic, wet) are deep, poorly drained organic soils consisting of muck, peat, or mucky peat. They are usually highly deficient in plant nutrients and often highly acidic. Most of these soils are saturated year-round and occur in marshes and low-energy swamps along some estuaries.
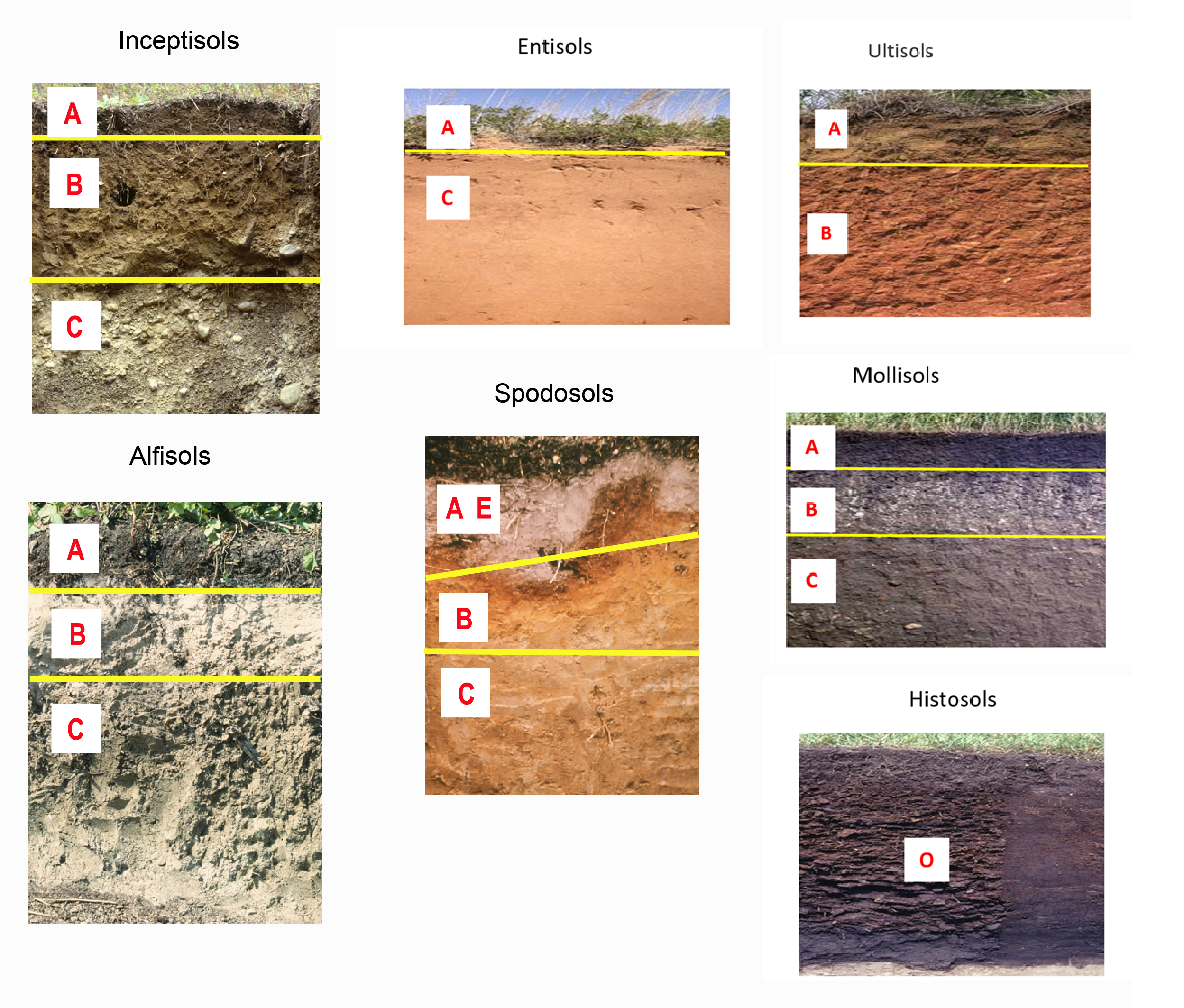
The following diagram shows general degree of weathering and soil development in different soil orders. Also shown are the general climate and vegetative conditions under which soils in each order are formed.[9] Virginia soil orders are indicated with boxes.
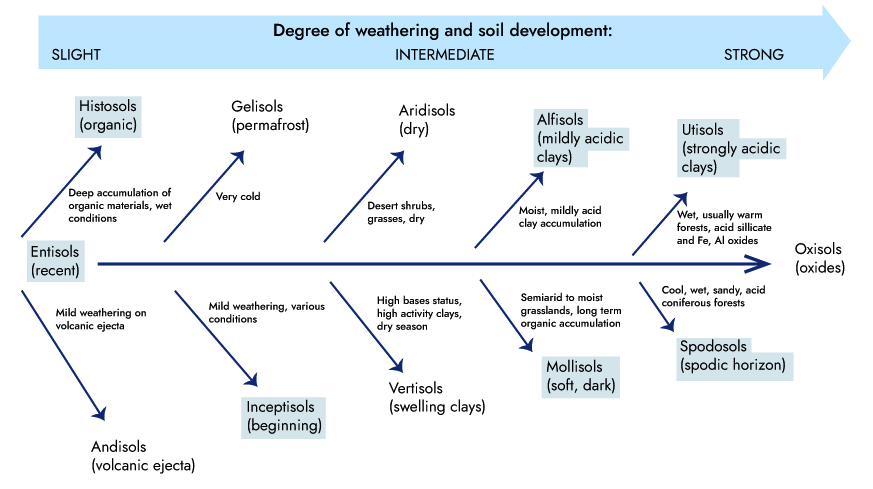
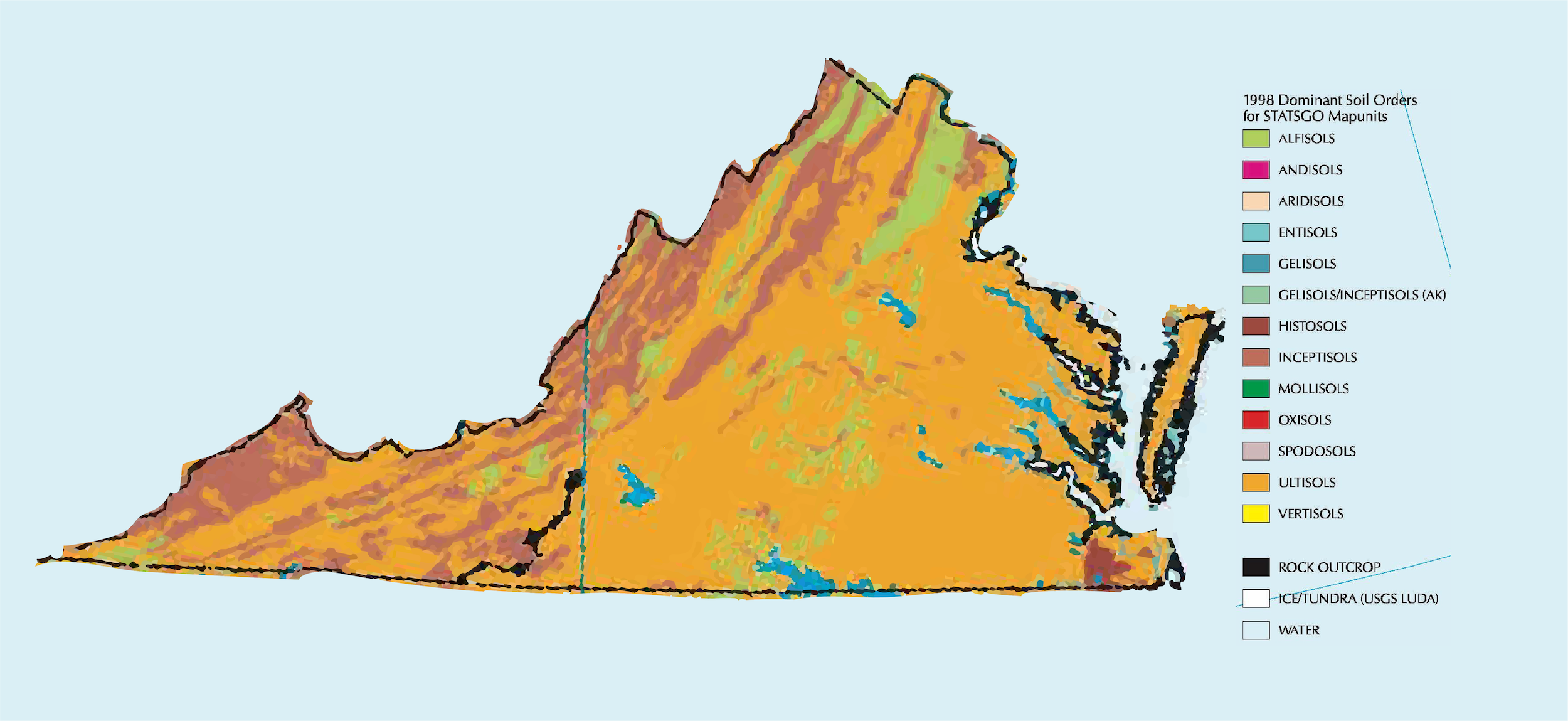
Interactive survey map allowing drilling down to specific addresses: https://casoilresource.lawr.ucdavis.edu/see/
Table 6-1 Distinguishing Factors of Virginia soils
Chart of Virginia soil orders
Soil Order | Characteristics of Horizon and Color | Chemical Composition | Tree Growth Requirements |
---|---|---|---|
Ultisols | ‘A’ is leached, pale
‘B’ has infertile clay accumulation. Red or yellow red due to insoluble iron oxides. |
‘A’ Acidic (H+, Al3+)
‘B’ Low in base cations: Ca2+, Mg2+, and K+ Base Sat. <35% pH ∞5.0 |
Dominant soil throughout the oak-pine range. Require inputs of lime and fertilizers. |
Alfisols | ‘A’ is brown to dark gray
‘B’ has moderately fertile clay accumulation. |
‘A’ Acidic: Al3+, Fe2+
‘B’ High in basic cations: Ca2+, Mg2+, K+, Na+ Cation sat. >35% pH ∞6.0 |
Deciduous forest of oak-hickory. |
Inceptisols | Weak, brown
’B’ horizon development. |
Varied
pH ∞6.0 |
Mixed or hardwood forest in the Eastern States. |
Entisols | No ‘B’ horizon development.
Light colors, often very sandy or gravelly. |
Varied
pH ∞7.0 |
Fertile in flood plains, others must be enhanced. |
Mollisols | Thick, dark, fertile, high carbon ‘A’ horizons. | High in basic cations: Ca2+, Mg2+, Na+, and K+
Base sat. >50% Very high in Ca2+ pH ∞7.0 |
Very fertile floodplain soils supporting sycamore, ash, hickory, cedar, and oak. |
Spodosols | ‘A’ is light-colored
sand. ‘B’ is very dark, accumulations of humus-Al, with or without Fe. |
Acidity: H+, Al3
Makes Organic Acids ‘B’ Low in basic cations: Ca2+, Mg2+, and K+) pH ∞5.0 |
Limited to acid-tolerant crops and orchards.
(Dogwood, Beech |
Histosols | Saturated, dark colored organic materials usually more than 20% organic carbon. | High Carbon content pH ∞4.0 |
(Red maple, black willow aspen, cottonwood, ashes, elms, swamp white oak, pin oak, tupelo and birches). |
No discussion is complete without mention of calcareous soils which contain an excess of calcium carbonate and have pH typically in the range of 7.2 and 8.5. Calcareous soils are found in dry calcareous forests in the Ridge and Valley area in the mountains of western Virginia. Calcareous fens and spring marshes, small-patch wetlands that developed over limestone or dolomite and are saturated by calcareous groundwater, are limited in Virginia to a few sites in carbonate rock districts of the Ridge and Valley area.[10] Trees are typically dominated by oaks, hickory, ash, and Eastern Red Cedar.
Coastal Plain Dry Calcareous Forests form a group of rare, deciduous (rarely mixed) forests and woodlands of fertile habitats over unconsolidated, calcareous deposits. In Virginia, occurrences are small and highly localized in two environmental situations: 1) steep, convex, south-facing slopes of dissected ravine systems and river-fronting bluffs of the inner Coastal Plain from southeastern Virginia north to Stafford County; and 2) steep cut-slopes bordering estuaries on the outer Coastal Plain. In the first setting, slopes have downcut into Tertiary shell deposits or limesands, producing circumneutral to slightly alkaline soils. In the estuarine settings, shell middens may provide the primary source of substrate calcium. The majority of documented stands are on The Peninsula near Williamsburg (James City and York Counties).[11]
Trees that tolerate calcareous soils include sugar maple (Acer saccharum), yellowwood (Cladrastis kentukea), Chinkapin oak (Quercus muelenberghii), elms (Ulmus spp.), American hornbeam (Carpinus caroliniana), European hornbeam (Carpinus betulus), redbud (Cercis canadensis), black walnut (Juglans nigra), sycamore (Platanus occidentalis), hophornbeam (Ostrya virginiana) and lindens (Tilia spp.).[12]
Effects of Land Utilization on Soil
Now that the naturally occurring types of soils have been discussed, what happens with the intervention of man: farming, silviculture, and urban and city development activities?
In Virginia there are large tracts of soils highly modified by humans, including soils of urban and suburban developments, landfills, transportation corridors, and mined lands. These soils may contain artifacts such as garbage or brick, concrete, or asphalt. They are typically higher pH than surrounding soils and may exceed pH 7.0. Other modified soils are either deeply excavated or deeply filled, with few to numerous rock fragments. Development is minimal like Entisols and Inceptisols, except where the soils are excavated versions of Ultisols and Alfisols. Trees are typically introduced and many are invasive.
When planting trees, if the soil quality is ignored the chances of growing the long-lived, large, healthy trees are greatly reduced[13]. A soil analysis and site history should be performed prior to plantings in order to provide the most appropriate growing conditions.
Note: For a detail soil analysis of the Virginia counties visit USDA NRCS, Soils.[14]
What has happened to our soil due to human intervention? “In 1630, forest covered 96% of Virginia’s land area. Over the first 230 years of European settlement much of the land was cleared for agriculture at one time or another, especially in the Coastal Plain and Piedmont. On poorer soils, attempts at cultivation were usually short-lived, and those areas reverted to forest. Thus, during this initial 230-year period of settlement, an average forest cover of 40–60% was maintained across Virginia (Scirvani 2003). Areas left in forest were cut for domestic uses and commonly subjected to free-ranging cattle and hogs (Woodward and MacDonald 1991; Martin and Boyce 1993). Soil depletion and erosion during this time were severe and led to massive sediment loading in floodplains and bottomlands. During the nineteenth century, accessible oak forests in the Ridge and Valley, Blue Ridge, and northern Piedmont were repeatedly cut for tanbark and processed into charcoal to fuel the furnaces of an active iron industry (Orwig and Abrams 1994; USDA Forest Service unpublished data). Most of the older forests remaining in the Piedmont and Coastal Plain at the time of the Civil War were destroyed to fill both armies’ prodigious needs for construction lumber and firewood. Natural, accidental, and intentionally set fires continued to burn forests and fields at irregular intervals throughout this era, since there were no effective ways of stopping or controlling them once started.”[15]
Agricultural activities associated with growing crops and raising livestock affects the physical and chemical composition of soils. Below depicts how plowing changes the soil horizons over time.
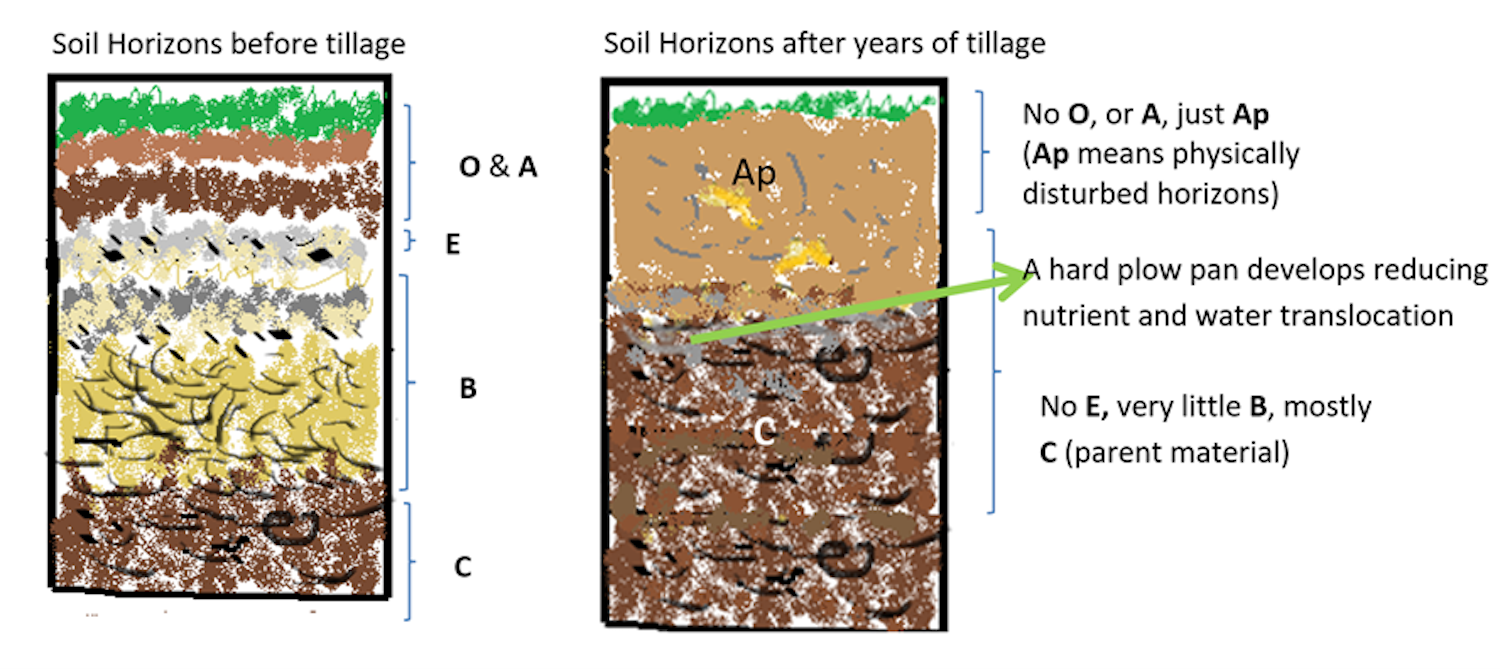
Plowing
Grassland soil into crop fields results in a release of nutrients that slowly declines, much like slash-and-burn agriculture. All tillage operations, including aeration and lifting, cause direct damage to soil macrofauna (moles, mice, earthworms…) and potentially expose them to new predators. Increased intensity of tillage is usually linked to disruptions in the habitat space for soil organisms and a decrease in the time the soil is covered by a growing plant (whether trees, crops, or weeds). There is evidence that the food web of soil organisms under farmed fields is less robust than that found under heaths and woodlands.
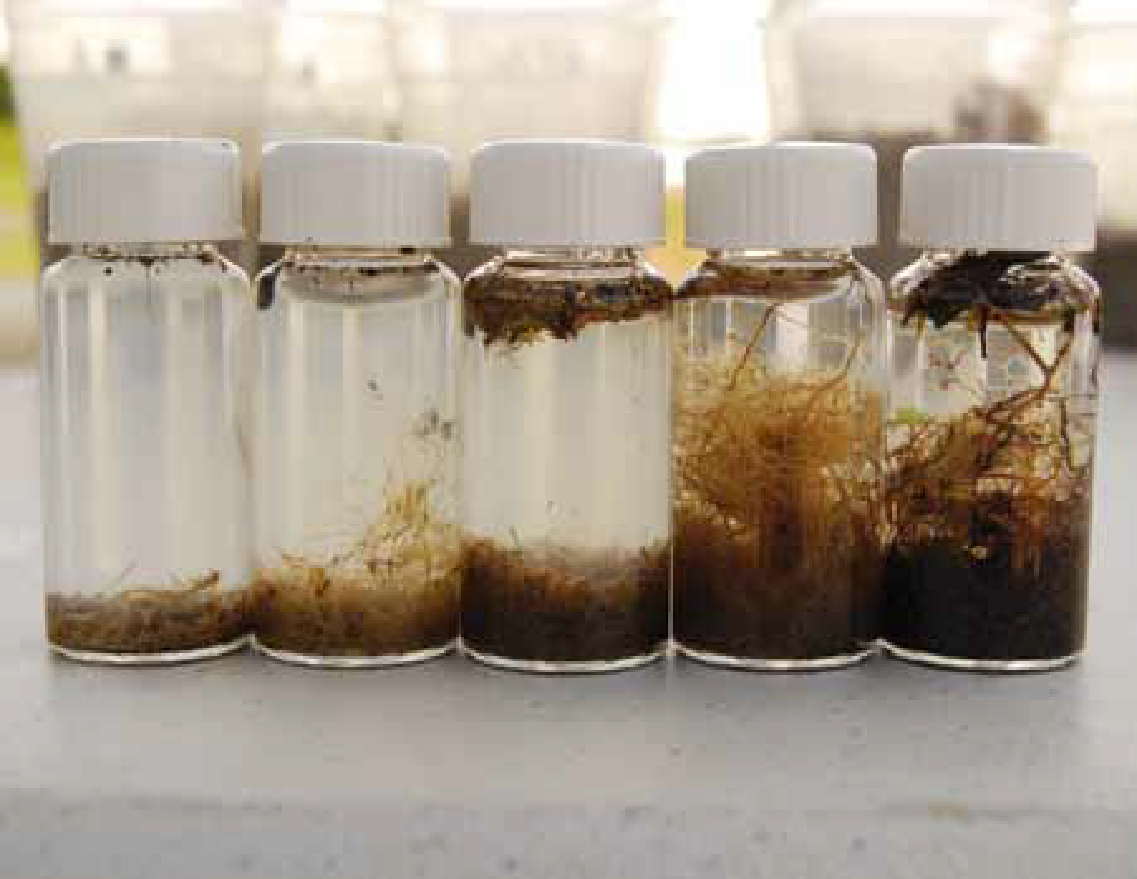
“Particulate organic matter such as this contains organic forms of nutrients which can be made available to plants through microbial decomposition processes. Vials to the left of center had increasing levels of tillage in the crop rotation while vials to the right of center were from un-tilled soils under permanent grass sod and forest. The vial in the center is from a continuous no-till field with annual crop rotation.” From Penn State “Managing Soil Health: Concepts and Practices”, 2012.[16]
Farmland or grasslands that are well managed have an “A” horizon soil with enough organic matter to supply plant nutrients, increases soil aggregation, limits soil erosion, and also increases cation exchange and water holding capacities, all of which are ideal for trees and other plants. Early growth of trees on former farmland may be greatly enhanced by treatments associated with the previous land use, such as fertilization or cultivation of an agricultural crop, and by post planting control of competing vegetation. One of the best known examples of the association of tree growth with previous land use is the “old-field effect” on the growth of loblolly pine (Pinus taeda) plantations in the southeastern United States. The effect was named when it was noticed that pine seedlings planted on old fields often grew faster than those planted between 1957 and 1963 on cutover sites. A similar phenomenon known as the “pasture effect”, that is, there is better tree growth on land previously in pasture than on sites previously in tree cover.[17]
Farmland used as pastures and feedlots where the trampling, pawing, and wallowing by hoofed farm animals disturb the soil and in some cases completely destroy the soil crusts. The most severe effect of trampling is the compaction of soils which damages plant roots. These changes may prevent plants from acquiring sufficient resources for vigorous growth and causes roots to become concentrated near the soil surface. Heavy livestock such as cattle compact soil structure and destroy vegetation on parts of a field that they tread most often. Physically damaged soil can be even more susceptible to the chemical and biological impact of feces and urine. Destruction of soil structure can be harmful because restoration of vegetation does not always occur spontaneously once the grazing animal is withdrawn.[18]
Forest soils, both natural and silvicultural, provide important functions:
- “Providing water, nutrients, and physical support for the growth of trees and other forest plants.
- Allowing an exchange of carbon dioxide, oxygen, and other gasses that affect root growth and soil organisms.
- Providing a substrate for organisms linked with vital ecosystem processes.
- Harboring root diseases and other pests.
- Affecting water quantity and quality”[19]
In undisturbed forest soils under broadleaf trees, autumn leaf falls provide abundant and rich humus which begins to decay rapidly in spring, just as the growing season begins. Where parent materials are sandy, evergreen vegetation tends to dominate the landscape.
“Healthy forest soils act like a giant sponge, absorbing precipitation, holding water against the force of gravity, and then slowly releasing it. In actively managed (working) forests, road construction, soil compaction, and organic matter removal from harvesting and site preparation can change the amount and timing of water flows and cause excessive soil erosion with the consequence of degrading water quality and fish habitat. Forestry access and timbering roads, particularly those built to older design standards or that are not maintained are regarded as the cause of most of the soil erosion and stream sedimentation coming from managed forests.”[20]
Timbering creates forest litter, including slash after logging. The litter ranges greatly in amount, size, nutrient content, and stage of decomposition.[21] Removing tree trunks may have little effect on site productivity, but “cleaning up” branches and foliage (i.e., slash) appears to have greater potential for nutrient removal than leaving them onsite. Decomposition after timber harvest frees mineral nutrients from organic matter, which increases mineral nutrient leaching until the site is revegetated.[22]
Forest fires, either natural or prescribed, heat the soil. Light, moderate, or intense fires affect the soil’s physical, chemical, and biological properties and the amount of organic matter destroyed is directly related to the intensity of the fire. Soil nutrients are either lost by volatilization, or are transformed into highly available ions by the burning. Nitrogen, for example, is easily volatilized and lost during burning. The nutrients not volatilized: calcium, magnesium, potassium, sodium, and phosphorus-are released as highly mobile ions which can be metabolized rapidly either by plants or microorganisms on the sites, or can be lost by erosion and runoff. The effect of soil heating on micro-organisms is less well understood but, micro-organisms are affected lethally at much lower temperatures than those necessary to change nonliving organic matter.[23]
Although fires, either natural or prescribed, do change the soil characteristics, bear in mind that many tree species depend on fire for growth (removes competition), maturation (opens canopy), and release of seeds, triggered, in whole or in part, by fire or smoke. Virginia trees that depend on fires are certain pine species such as longleaf pines.
Timbering and burning can also affect the hydrology of cleared forest land, especially sloped areas. When tree roots are left to rot or are burned a “soil pipe” often occurs. This is like the “lost wax effect”. Once the wood is gone a “pipe” remains. The presence of soil pipes can affect how water drains or flows through the soil and plays a role in soil erosion and hillslope stability. The presence of soil pipes can cause retained water to drain faster than normal, potentially causing flooding during the wet season and empty streams in the dry season.[24][25]
Soils produced by coal mines tailings, power plants fly ash and household and industrial waste sites are known as mined or anthropogenic soils.
“The amount of coal refuse in the Appalachian coal fields is difficult to estimate, but active disposal facilities cover thousands of acres, and abandoned refuse piles dot the landscape in almost every major watershed. A vigorous plant community can reduce water and oxygen erosion. Establishment and maintenance of permanent vegetation on refuse, however, is complicated by physical, mineralogical, and chemical factors.”[26]
“Because of the inherently low fertility of refuse, vegetation establishment requires the addition of nitrogen, phosphorus, and potassium fertilizers. Currently, very little has been documented about the use of woody plants for the reclamation and revegetation of coal refuse. Industry experience indicates that black locust (Robinia pseudoacacia L.), white pine (Pinus strobus), and red pine (Pinus resinosa) can be successfully direct-hydroseeded onto conditioned refuse. “[27]
Reclamation of chemical (toxic) waste sites (brownfields) requires time and is costly. The contaminated soils must be removed and new soil brought in. Tree growth can occur and helps absorb the contaminants and heavy metals. Heavy metal residues do not usually affect tree growth but trees sequester and systemically distribute the heavy metals. Animals may be poisoned by eating parts or fruits of the trees. Of course, herbicidal waste will prohibit plant growth and must be neutralized.
Urban soils in “built environments” can be challenging to manage for non-invasive native tree production. The status of urban soil can be natural, anthropogenic, compacted, horizon disturbed, or a combination of all. The challenge is to provide an urban environment that functions like the natural environment. It is important to note that some trees are adaptable to a fairly wide range of environmental conditions while others have a narrow range in which they will grow well. All trees will grow well under near optimal conditions with a pH of 6.8 and consistently moist but well drained soil. However, we rarely find these conditions in the urban environment. Most urban soils have a higher pH (from near neutral to alkaline) than surrounding rural areas due to limestone-containing materials in the street environment.[28]
“The type of soil that a tree or shrub grows in can affect its nutrient needs. Soil texture and soil structure influence the amount of water, air, and nutrients held in the soil for plant use. Clay soils can be nutrient rich, but have a large amount of fine particles that tend to compact and restrict water and air movement. Sandy soils drain well, but contain many coarse particles that have little capacity for storing water, air and nutrients. Organic material can be thoroughly mixed into soils with high clay or sand contents to help improve soil structure. Repeated applications may be needed depending on the amount applied and the stage of decomposition or type of organic matter used. Organic material should be mixed into the soil up to several years before trees are installed to obtain maximum benefit.”[29]
“Characteristics of soil in any urban area depend on many things. They depend on how deep the site has been excavated during construction and if new materials were brought in and mixed with the original soil materials. They depend on the properties of the original natural soil and the past uses of the site. Often the topsoil is removed from the site prior to construction and may or may not be returned to the site. After excavation, subsoil may be placed as fill over topsoil. Changing the order of the soil layers or mixing the topsoil and subsoil can alter soil properties. These variables make predicting soil behavior difficult in urban areas.”[30] Tree root growth occurs mainly in the ‘A’ horizon which normally contains the most nutrients. If the horizons have been mingled or removed the planter must restore their beneficial properties.
Examples of the Factors That May Affect the Productivity of Urban Soil
- Little or no addition of organic matter.
- Artifacts that disrupt water movement.
- Elevated salt content.
- Interrupted nutrient cycling and modified activity of micro-organisms.
- High soil temperatures that increase the rate of chemical reactions.
- Generally higher pH values resulting from additions of cement, plaster, and road salts.
- Lateral (sideways) subsurface water flow resulting from compacted layers.
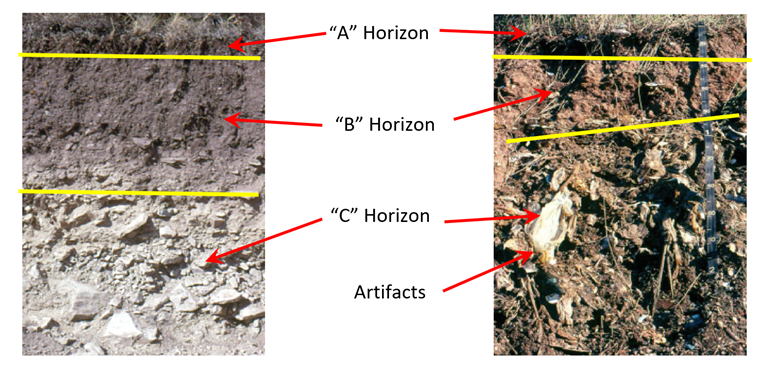
Urban or city soils containing artifacts are called “Human-altered and human-transported” soils . “They are characteristically heterogeneous and often suffer from [reduced organic carbon], excessive compaction, excessive artifact content, diminished biological activity, and increased run off [reduced infiltration rate]due to surface crusting or water repellency. They generally have elevated pH, exchangeable bases, and carbonate content. Levels of organic C+4, N-3 and P-3 tend to be very low in recently deposited human transported material. Unaided, urban soils may take 30-100 years to reestablish properties similar to those of natural soils.”[31]
The pictures below show disturbed urban soils in eastern Virginia.
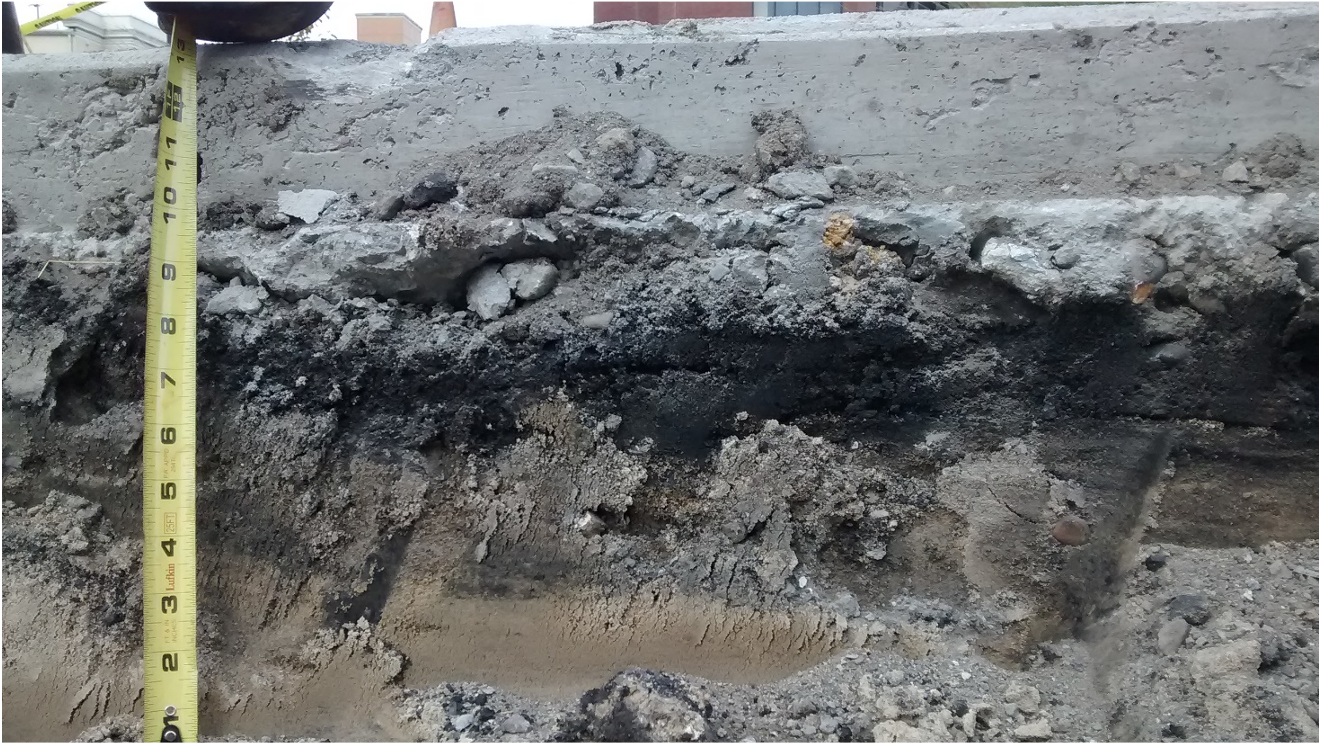
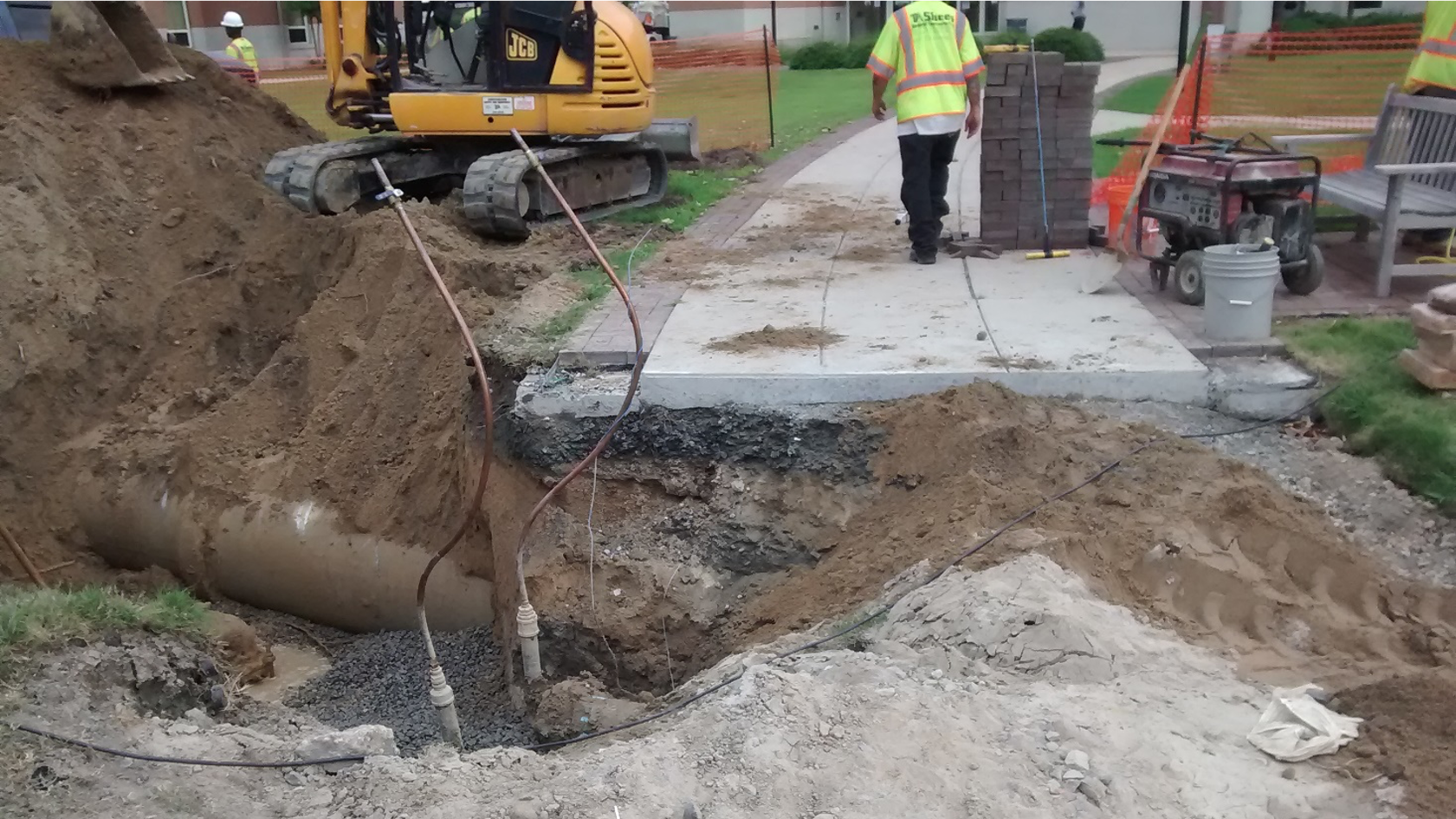
Artifacts often occur in soil altered or transported by humans. Examples exist in table 6-2:
Table 6-2 Artifacts in virginia soils
Asphalt | Concrete | Metal | Mechanically abraded rock fragments |
Brick | Fertilizers | Paper | Mining and milling waste |
Cardboard | Glass | Plastic | Combusted coal by products |
Carpet | Heavy metals | Pesticides | Wood products |
Cloth | Midden | Rubber | Salts |
Horizon disturbance refers to the change of the natural soil horizon arrangement in the soil profile. Most of the changes occur by the removal of the top soil, dumping of construction waste, or compaction of site prior to tree planting. “Poor quality subsoil with fine texture or high clay content is often brought to the surface or used as fill soil.”[32]“Tree growth enhancements may include overcoming physical and chemical root restrictions, water supply, and drainage to allow tree growth. The soil may require amendment to provide the nutrients to balance soil fertility and acidity (pH), and reduce the likelihood of contamination or disease problems.”[33]
Compaction refers to the change in soil bulk density which is dependent on soil particulate matter size, moisture content of the soil, and the type or weight applying the pressure (human or vehicular traffic, construction equipment, and vibrations). Soil crusting occurs. “As soil particles are pressed together, root penetration, water infiltration, and drainage rates are reduced.”[34]Compaction can be reduced by mechanically deep tilling, vertical aeration (coring), or vertical mulching (digging trenches and backfilling with amended or original soil).[35] Care must be taken not to till the soil when wet or moist.
“Soil Profile Rebuilding is an appropriate soil restoration technique for sites where topsoil has been completely or partially removed and subsoil layers have been compacted (graded and/or trafficked by equipment) such as the staging areas near building or road construction sites. It may also be used with some modifications if topsoil is present. This is not an appropriate technique in sites with surface compaction only (6 inches or less), although this situation is rare on construction sites. This technique is not appropriate within the root zones of trees that are to be protected since it will break apart existing tree roots. Soil Profile Rebuilding can improve physical and biological characteristics of soil to allow for revegetation. It does not address soil chemical problems, soil contamination from heavy metals, pathogens, excessive debris or gravel.”[36]
“One new tool for urban tree establishment is the redesign of the entire pavement profile to meet the load-bearing requirement for structurally sound pavement installation while encouraging deep root growth away from the pavement surface. The new pavement substrate, called ‘structural soil’, has been developed and tested so that it can be compacted to meet engineering requirements for paved surfaces, yet possess qualities that allow roots to grow freely, under and away from the pavement, thereby reducing sidewalk heaving from tree roots.”[37]
Manmade Climate Change, Saltwater Intrusion, and Ghost Forests
At the beginning of the chapter the effects of climate and geologic factors were presented as the elements producing soil over millions of years. For centuries, man has made composition changes through agricultural and industrial activities. In the last 200 years there has been a rapid decline in coastal forests due to manmade climate change. Obvious change agents are sea level rise and saltwater intrusion into the eastern part of the state, resulting in increased soil salinity that kills trees, increases marshland, and creates ghost forests.
The pumping of fresh water from underground aquifers for agriculture and urban needs leads to saltwater intrusion. The inland water aquifers are gradually being affected all the way up to the natural fall zone between the piedmont and the coast. If water is pumped out faster than nature replenishes it, the water table levels decrease allowing salt water to fill the vacancy as shown in Figure 6-13.
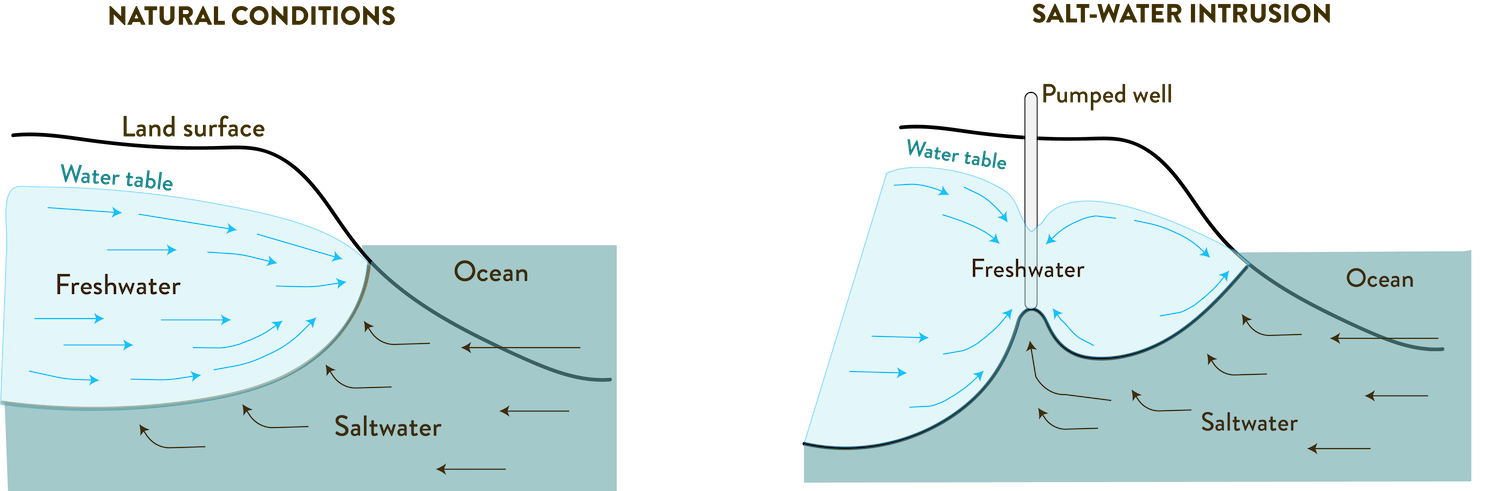
The increasing salinity on the Atlantic Coast is due to multiple factors. The melting of the ice caps, the change in the gulf stream flow, increasing frequency of storms pushing sea water inland, gradual sinking of the shoreline due to geologic movements, the reduction of the underground aquifer water supplies, and inland droughts reducing the amount of fresh water flowing to the ocean all affect water salinity levels.
As salinity increases, the most sensitive trees succumb. The leaves and needles dehydrate, turn brown, and die. The foliar damage decreases the tree’s ability to photosynthesize which gradually reduces root energy storage and results in death. Oak, hickory, and other hardwoods die first, then cedars, and finally loblolly pines. When the older trees die there are no replacements. Seeds and seedlings are more sensitive than older trees to the salinity increase; therefore, there are no undergrowth replacement trees.
Currently there are no mechanical means to prevent sea level rise or saltwater intrusion. Active reforestation research using more salt tolerant hybrids of coastal forest trees offers hope. Chapters Seven and Eight offer some more discussion.
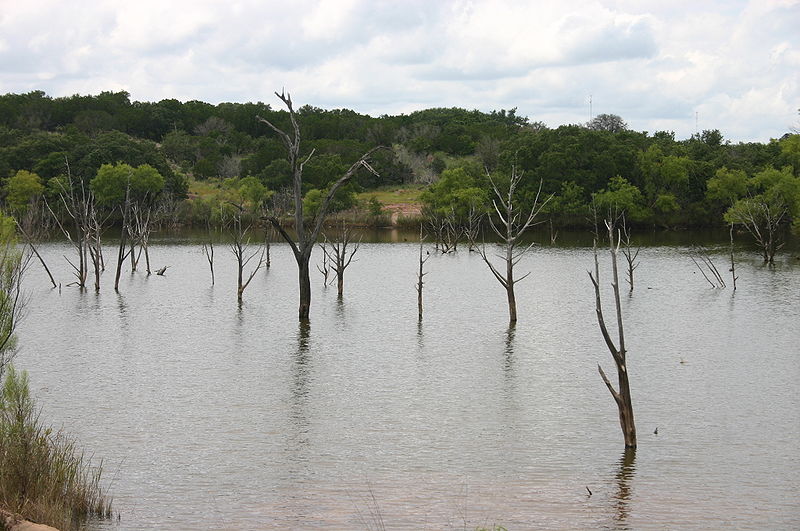
Tree and Shrub Fertilization
Woodland trees, with their duff cover and canopy coverage, rarely need fertilizing if their environment has remained the same for an extended time, but trees in urban areas do not have the environmental advantages of woodland trees. Topsoil and the vegetative cover have often been removed while various mechanical injuries have resulted from digging for irrigation and utility lines or for buildings and pavement. There are many questions to consider when assessing the ability of a soil to grow trees. What amount of compaction is restricting root growth? Are tree roots in sites of limited soil volume? What contaminants are in the soil? Is the tree sharing root space with other trees? What is its nutrient content?
Sometimes fertilization can also stress a tree, so determining an urban tree’s need for fertilization is not a lightly-made decision.[38] All fertilizers are salts which can increase the salinity of the soil. Fertilization may also decrease a tree’s resistance to sucking and chewing insects because it’s energy is spent in growth at the expense of defensive chemicals.[39] Overfertilizing a soil can also lead to excessive vegetative growth on a tree at the expense of flower and fruit production, or release of excess nutrients to deep percolation or surface waters. Misuse of fertilizers may also add to other environmental issues which may need remediation to clean up subsequent problems.
Tree care professionals research a tree species and know how it is supposed to look when it is healthy and vigorous in order to recognize symptoms that indicate when something is not normal for that species. Visual inspections of a leaf’s symptoms can lead to multiple suggestible causes including insect damage, environmental stress, pathogen issues or a combination of stressors, but soil and foliar analyses can definitively determine a nutrient deficiency either in the soil or in the leaves. Soil test kits are readily available from Virginia Tech and give essential nutrient amounts, except nitrogen, in the soil, along with the soil sample’s pH.
Once a nutrient deficiency has been corrected, maintenance fertilization can replace nutrients lost to natural nutrient cycling or to restore balance when nutrients are lost to tree litter removal. Growth promoting fertilizer[40][41] may be needed for container trees or young trees in rapid growth stages to encourage vegetative growth, flowering, and/or fruit production depending upon the nutrients been applied. Also keep in mind that young trees use more N than more mature trees.
Soil Tests
In addition to the availability of the essential nutrients in the soil, soil tests also determine pH which measures the number of free hydrogen ions (H+) in the soil solution. pH is measured on a scale of 1 – 14 with 7 being neutral. Readings less than 7 indicate acid soils and readings greater than 7 indicate basic or alkaline soils. Areas of high rainfall, as befits Virginia, tend to have acid soils due to basic elements such as Ca, Mg, K, and Na being washed away. If pH is too low for the tree species being considered for an urban landscape, apply lime (calcium carbonate) according to soil test recommendations to raise the pH. If the pH is less than 5.0, use a non-ammoniacal source of nitrogen to raise the pH. If pH reading falls between 5.0 and 7.2, most other fertilizers can be used. The pH range most conducive to temperate trees and shrubs is 5.5 – 6.5.[42] Refer to “Virginia Tech Publication 430-027 Trees and Shrubs for Acid Soils” for recommended trees and shrubs for various acid soils.[43]
Areas with poor rainfall have basic soils. If pH is greater than 7.2 or is too basic for the tree species being considered, then apply elemental sulfur or fertilizers containing ammonium (NH4+). Aluminum sulfate can also acidify soil but it is generally not recommended because it adds Aluminum cations to the soil. Aluminum, a nonessential element which tends to be available in soils, is toxic to trees and becomes more readily uptaken by trees as pH decreases.
Reducing pH significantly in calcareous soils is unrealistic.[44] These soils inhibit nutrient uptake of many of the essential nutrients especially iron, zinc, manganese, and boron; however, they have high levels of magnesium and calcium carbonates which are readily available for uptake by trees.[45]Fertilizers with ammonia volatize easily in high pH soils.
Saline soils have excessive sodium content and can hinder growth and development of trees than are not salt-tolerant. In areas with 20 inches or more rainfall annually, saline soils typically are not a problem. Saline soils can be caused when areas are irrigated with water containing dissolved salts, use of de-icing salts, encroachment of seawater, over fertilization, and brackish water encroachment.
Salt-tolerant plants or shrubs, which divert sodium ions away from a tree’s absorbing roots, may be planted within a tree’s dripline. The soil can also be flushed with large amounts of quality water. Refer to “Virginia Tech Publication 430-031 Trees and Shrubs That Tolerate Saline Soils and Salt Spray Drift” for a list of salt-tolerant trees and shrubs.[46]
“Soil pH also influences the composition/activity of microorganisms. Generally, the activity of fungi increases at lower pH and the activity of bacteria increase with rising pH.”[47]
Fertilizer Types
Recall that a fertilizer’s analysis is always written in the form N – P – K which gives the rate of nitrogenous fertilizer, the amount of phosphate (P2O5), and the amount of potash (K2O). While all of the nitrogenous form is available to the plant, only 43% of phosphate is actual phosphorus content, and 83% of potash is actual potassium.[48]While a complete fertilizer contains all three of these essential elements, an incomplete fertilizer is missing one or two of these elements.
Fertilizers may also be categorized by their mode of action, either fast- or quick-release and slow- or controlled-release. Although fast-release fertilizers are soluble immediately, they also tend to be more prone to leaching and have a higher potential for phytotoxicity. Because of these negative characteristics, they are usually not recommended.[49] Slow- or controlled-release fertilizers are more soluble over time, have few phytotoxicity effects, and can be applied at higher rates with fewer applications or at lower rates over multiple applications. The coatings of slow-release fertilizers slow down the rate of nutrients released to plants. Water, heat, and microbial activity decompose various slow-release forms. Of slow-release and controlled-release forms, controlled-release forms actually control the rate at which nutrients become available to the plants whereas slow-release forms slow the process of nutrient availability from that of quick-release forms.
Fertilizer forms may be either organic or inorganic. While the chemistry definition of organic refers to a compound containing carbon, another definition says that organic forms come from once-living organisms,[50]which is the definition followed here. Keep in mind that trees uptake inorganic ions regardless of the source of nutrition being organic or inorganic. The applicator is the determinant of the form being used.
Organic forms may be composted manure, treated sewage sludge, fish emulsion, bat guano, or compost. These may be applied either in liquid or dry formulations and may be available in the industry in either natural or synthetic forms. Organic forms are slow-release fertilizers that do not leach readily from soil due to their slow conversion to inorganic ions that are subsequently taken up by plants.
Inorganic forms have higher concentrations of essential elements, are water soluble, and are less affected by soil temperature than organic forms. Inorganic formulations may be either liquid or dry. Inorganic sources of iron, zinc and manganese may be applied in chelated forms, which are highly soluble yet hold metal ions in solution, to either the soil or the foliage.[51]
Fertilizer Application Methods[52]
Whether fertilizers are liquid or dry formulations, they may be effectively broadcast as long as they are applied over the rooting zone as a surface application. Some issues may arise with the broadcast method. If the soil surface is covered with turf or an organic mulch, the essential nutrients, especially phosphorus, in the fertilizer may not work their way into the root zone without being taken up by turf or fixed in the mulch. Due to runoff potential, broadcasting fertilizers is not recommended on slopes. Broadcasting is a good method for open grown trees with little or no understory vegetation.
The most common method used by commercial arborists is sub-surface, which works well with liquid or dry formulations also. This is the best method to use for trees with competing understory vegetation. Typically, a grid of holes, 4 – 8 inches deep and 12 – 36 inches apart, is dug between the trunk and the dripline and the same amount of fertilizer is applied to each hole. This is a standard method for application of amendments also. Soil injection is another form of sub-surface application but is costly and needs specialized equipment.
Before you dig, contact Miss Utility at va811.com or dial 8-1-1 for utility lines. Homeowners are responsible for irrigation lines, which are not located by Miss Utility.
Trunk injection is used to correct a micronutrient deficiency such as iron. The preferred time of year is during spring or early summer after leaves have fully expanded, with one application annually. Shallow holes are made with drill bits into the flare of the tree, which presents the dilemma of wounding the trunk; however, annual applications via trunk injection are discouraged. Injection is not efficient with macronutrients. Trunk injections for fertilizers are considered to be a controversial practice among many professionals in the industry because it treats the symptoms and not the underlying cause.
When soil applications have limited success with nitrogen, iron or zinc, foliar application of fertilizers may be considered. A liquid formulation of fast-release fertilizer is sprayed onto young leaf surfaces where uptake is the highest. Entry into the leaves is via stomata but a leaf’s waxy or oily surface will impede entry.
Rate of Application
Because ANSI standards change periodically, it is best to access both the standards and related Best Management Practices for Tree and Shrub Fertilization. Currently the 3rd edition 2013[53] is available. For annual maintenance fertilizer, 2 – 3 pounds of slow release N per 1000 ft2 in the temperate zone are recommended. While fast release fertilizers are not recommended, 1 – 2 pounds of fast release N per 1000 ft2 may be used for maintenance when slow release fertilizers do not meet objectives.
For corrective fertilization of nitrogen deficiency or when growth promotion is encouraged, the typical rate is 3 – 6 pounds of slow release N per 1000 ft.2
Timing of Application
Early in the growing season, plants are using stored reserves of essential nutrients. The reservoir peaks at bud break and diminishes as the leaves mature; however, the time of maximum nutrient uptake is throughout the growing season from after bud break in the spring until leaf color change in the fall.[54]Adequate soil moisture is necessary for nutrient uptake.
Fall application typically does not predispose a healthy tree to winter injury. Stressors such as topping or shearing, excessive nitrogen levels that promote vegetative growth late in the season, non-native tree species, and an indeterminate growth habit may cause injury with autumn fertilizer applications.[55]
Environmental issues can dictate timing of application. Avoid application before or during heavy rains to avoid run-off. Application during drought is not recommended because absorption by plants is minimal and fertilizer salts may build excessively.
It is not recommended to apply fertilizer during transplanting when a transplant’s root system is compromised unless the soil is proven to be nutrient-deficient. In that case, incorporate the fertilizer into the backfill soil or beyond the root ball. Although phosphorus is usually available in sufficient quantities in soils, urban soils may lack this nutrient and need to be supplemented.[56]
Table 6-3 Organic Soil Amendments
Common Name | Analysis/Composition | Notes |
---|---|---|
Bat guano | 10--3--1; 3--10--1 (variable) | Decomposed/dry guano; bioremediation of toxic soils |
Blood meal | 12.5--1.3--0.7 | Expensive; quick-acting N; can burn plants; lasts 4 months |
Bone meal | 3--20--0 + 20%-30% Ca | Quick P; Lasts 12 months or longer |
Clodbuster or Leonardite | 15% humic materials; 15% humic acid | Helps make nutrients available to plants; lasts 12 months |
Composted manure | Variable; 1-2--0.5-1.5--1-1 | Slow-release |
Cottonseed meal | 3-6--2--1-2 | Lasts 4-6 months; may contain pesticide residues |
Fish emulsion | 4--4--1 | Boosts seedlings |
Fish meal | 10.5--6--0 | Lasts 6-8 months |
Greensand | 0--1.5--6-7 | Glauconite (iron potassium silicate); slow-release; insoluble; loosens heavy clay soils |
Kelp meal & liquid seaweed | 1--0--1.2 | Concern about contamination from Increasing sea pollution; lasts 6-12 months |
Soybean meal | 6--2--2 | From soybean oil processing |
Treated sewage sludge | 4-5--2--0.32; Milorganite | Biosolids; slow-release; promotes rapid timber growth |
Wood ashes | 0-10% K2O + 5% Mg + 50% CaCO3- | Increases pH; water soluble; lasts 12 months or longer |
Fertilization of trees and shrubs has its place in a well-managed tree care program. People in the tree care industry, such as certified arborists and tree stewards, realize that a need for fertilization must exist before application to avoid instances of misapplication. Once the need is established and a specific fertilizer area is determined, consider the tree species, its growth phase, the health of the tree, and soil conditions. In addition to attaining a certified arborist designation through International Society of Arboriculture, an applicator can also attain the Nutrient Management Certification which is offered by Virginia Department of Conservation and Recreation.[57]
Contracts must clearly state the objective(s) wanted to achieve an established goal. Then a soil analysis is needed to determine nutrient needs along with the fertilizer application. The exact application area and the method of application are also stated.
Table 6.4 Fertilizers
Chemical Name | Common Name | Composition | Notes |
---|---|---|---|
Ammonia gas | NH3 | 82-0-0; pH 11-12 | |
Ammonium | NH4+ | Decreases pH | |
Ammonium nitrate | (NH4)NO3 | 33.5-0-0; decreases pH; explosive | |
Ammonium phosphate | NH4P2O5 | 16-20-0; often with 13% S | |
Ammonium sulfate | (NH4+)2SO4 | 21-0-0; decreases pH; 24% S | |
Calcium carbonate | Limestone; calcite | CaCO2 | Increases pH; 40% Ca |
Calcium carbonate w/magnesium | Dolomitic limestone | CaCO2MgCO2 | Increases pH; 2-13% Mg |
Calcium chloride | CaCl2 | Deicer | |
Calcium hydroxide | Hydrated lime; slaked lime | Ca(OH)2 | Increases pH; expensive; caustic; pH>12 |
Calcium nitrate | Ca(NO3)2 | 15.5-0-0; increases pH; 19-22% Ca; pH 5-7 | |
Calcium oxide | Quicklime; burned lime | CaO | Increases pH; expensive; difficult to handle; releases heat when added to water |
Calcium sulfate | Gypsum | CaSO4 | No pH change; 29% Ca, 23% S |
Hydrogen carbonate | Bicarbonate–historical name; not bicarbonate of soda | HCO3- | Increases pH |
Hydrogen ion | H+ | When free in soil solution, then pH decreases | |
Hydroxide ion | OH– | When free in soil solution, then pH increases | |
Iron sulfate | Ferrous sulfate | FeSO4 | No NPK source; 20% Fe, 11% S |
Magnesium sulfate | Epsom salts | MgSO4 | No pH change; 10% Mg, 13% S |
Nitrate | NO3 – | Preferred form of N uptake by plants | |
Phosphate | P2O5 | Form found in fertilizers | |
Phosphoric acid | H3PO4 | Decreases pH in water | |
Potash | K2 O | Form found in fertilizers; mined product; any K compound | |
Potassium chloride | Muriate of potash | KCl | 0-0-60; no pH change |
Potassium nitrate | Salt peter | KNO3 | 13-0-44: increases pH 7-10 |
Potassium sulfate | Sulfate of potash (SOP) | K2 SO4 | 0–0–48-53; no pH change; 17-18% S |
Sodium nitrate | NaNO3 | 16-0-0: increases pH; 26% Na | |
Sulfur, elemental | S | Lowers pH; nonsoluble; use gypsum | |
Triple superphosphate | TSP | Ca(H2PO4)2 | 0-45-0; contains no gypsum; 15% Ca |
Urea | CO(NH2)2 | 45-0-0, 46-0-0, decreases pH; not considered an organic fertilizer | |
Urea formaldehyde | UF | 38-0-0; controlled release | |
Urea-ammonium nitrate | UAN or URAN | 28-32% N; liquid |
Review Questions
- What are 3 physiographic reasons tree species populations of the Appalachian slopes are usually different from the Coastal plains?
- Moisture retention contributes to successful tree establishment. What 2 soil elements affect retention?
- Soil Orders are usually differentiated by two or more ____________ & ____________ characteristics.
- In what horizons do most tree roots grow?
- Once disturbed, how does horizon displacement affect root growth and how long does it take to equilibrate the physical and chemical properties of soil?
- Microflora is essential to root nutrient adsorption. Explain this mycorrhizal symbiotic relationship.
- When planting trees, it is important to know prior land utilization. How do silviculture, farming, and industrial waste affect soil properties?
- Urban soils pose many problems to overcome when planting trees. Name 3 common obstacles.
- Name the primary limiting nutrient for woody plant growth and development.
- Name three situations when saline soils exist.
- True or False: Slow-release fertilizers actually control the rate at which nutrients become available to plants.
- True or False: Trunk injection of nutrients is the best way for trees to quickly receive the nutrients they need.
- The time for maximum nutrient uptake in trees is throughout the growing season between ___________________ in the spring and ___________________ change in the fall.
- True or False: Applying fertilizer to trees during transplant is a recommended practice.
- What must be true before a fertilizer program is recommended?
- True or False: Fall application of fertilizer typically does not predispose a healthy tree to winter injury.
- Schneider, C. (2014). Encouraging Urban Tree Growth with Organic Soil Amendments. Soil Science Society of America. www.soils.org ↵
- Gilman, E. (1997). Trees for Urban and Suburban Landscapes. Delmar Publishers. ↵
- Natural Resource Conservation Service. (2017). Published Soil Surveys for Virginia. https://www.nrcs.usda.gov/wps/portal/nrcs/surveylist/soils/survey/state?stateId=VA ↵
- Flsk, S.V. (2015). Farming Lessons from Mother Nature. Soil Science Society of America. https://www.soils.org/discover-soils/story/farming-lessons-mother-nature ↵
- Natural Resource Conservation Service. (2017). Published Soil Surveys for Virginia. https://www.nrcs.usda.gov/wps/portal/nrcs/surveylist/soils/survey/state?stateId=VA ↵
- School of Forest Resources & Conservation UF/IFAS. (2017). Forest Soils and Landscapes. dendro.cnre.vt.edu/forestbiology/htmltext/chapter7new.htm ↵
- . Natural Resource Conservation Service. (2017). Published Soil Surveys for Virginia. https://www.nrcs.usda.gov/wps/portal/nrcs/surveylist/soils/survey/state?stateId=VA ↵
- . Natural Resource Conservation Service. (2017). Published Soil Surveys for Virginia. https://www.nrcs.usda.gov/wps/portal/nrcs/surveylist/soils/survey/state?stateId=VA ↵
- Brady, N., Wei, R. (2007). The Nature and Properties of Soils (14th ed.). Prentice Hall. ↵
- Flemin, G.P., Patterson, K.D., Taverna K. (2017). The Natural Communities of Virginia: a Classification of Ecological Community Groups and Community Types. Virginia Department of Conservation and Recreation, Division of Natural Heritage, Richmond, VA. http://www.dcr.virginia.gov/natural-heritage/natural-communities/ ↵
- Flemin, G.P., Patterson, K.D., Taverna K. (2017). The Natural Communities of Virginia: a Classification of Ecological Community Groups and Community Types. Virginia Department of Conservation and Recreation, Division of Natural Heritage, Richmond, VA. http://www.dcr.virginia.gov/natural-heritage/natural-communities/ ↵
- Flemin, G.P., Patterson, K.D., Taverna K. (2017). The Natural Communities of Virginia: a Classification of Ecological Community Groups and Community Types. Virginia Department of Conservation and Recreation, Division of Natural Heritage, Richmond, VA. http://www.dcr.virginia.gov/natural-heritage/natural-communities/ ↵
- Urban, J. (2008). Up by Roots. ISA. ↵
- Reiter, E. (2019). A Multidisciplinary Approach to Conservation. Virginia Cooperative Extension. https://www.pubs.ext.vt.edu/content/dam/pubs.../2910-1417_pdf.pd ↵
- Natural Resource Conservation Service. (2017). Published Soil Surveys for Virginia. https://www.nrcs.usda.gov/wps/portal/nrcs/surveylist/soils/survey/state?stateId=VA ↵
- Penn State Extension. (2012). Managing Soil Health: Concepts and Practices. https://extension.psu.edu/managing-soil-health-concepts-and-practices ↵
- Murray, M., Harrington, C. (2017). Yield Comparison of Three Douglas-Fir Plantations on Former Farmland in Western Washington. USDA Forest Service, Forestry Sciences Laboratory. https://www.fs.fed.us/pnw/olympia/silv/publications/opt/341 ↵
- Franzluebbers, A., Stuedemann, J. (2017). Soil Organic Matter Stratification with Depth under Pastures in the Southern Piedmont USA. USDA. https://www.ars.usda.gov/research/.../publications-at-this-location ↵
- Helgerson, O., Miller, R. (2008). Keeping Your Forest Soils Health and Productive. Washington State University Extension. https://www.fs.usda.gov/treesearch/pubs/30437 ↵
- Helgerson, O., Miller, R. (2008). Keeping Your Forest Soils Health and Productive. Washington State University Extension. https://www.fs.usda.gov/treesearch/pubs/30437 ↵
- Helgerson, O., Miller, R. (2008). Keeping Your Forest Soils Health and Productive. Washington State University Extension. https://www.fs.usda.gov/treesearch/pubs/30437 ↵
- Helgerson, O., Miller, R. (2008). Keeping Your Forest Soils Health and Productive. Washington State University Extension. https://www.fs.usda.gov/treesearch/pubs/30437 ↵
- Helgerson, O., Miller, R. (2008). Keeping Your Forest Soils Health and Productive. Washington State University Extension. https://www.fs.usda.gov/treesearch/pubs/30437 ↵
- Kumar, S., Anderson, S., Udawatta, R. (2008, December). Agroforestry and Grass Buffer Influence on Macropore Measured by Computed Tomography under Grazed Pasture Systems. Soil Science Society of America Journal. 74(1), 203-212. www.soils.org ↵
- Maderick, R., Gaynon, S., Makuch, J. (2006). Environmental Effects of Conservation Practices on Grazing Lands. Agriculture Library ARS-USDA. https://www.ars.usda.gov/ARSUserFiles ↵
- Daniels, W., Stewart, B., Zipper, C. (2000). Reclamation of Coal Refuse Disposal Area. Virginia Cooperative Extension. pubs.ext.vt.edu/460/460-131/460-131_pdf.pdf ↵
- Daniels, W., Stewart, B., Zipper, C. (2000). Reclamation of Coal Refuse Disposal Area. Virginia Cooperative Extension. pubs.ext.vt.edu/460/460-131/460-131_pdf.pdf ↵
- Bassuk, N., Curtis, D., Marranca, B.Z., Neal, B. (n.d.) Recommended Urban Trees: Site Assessment and Tree Selection for Stress Tolerance. Urban Horticulture Institute, Department of Horticulture, Cornell University. http://www.hort.cornell.edu ↵
- Trozzo, K., Munsel, J., Chamberlain, J. (2012). Native Fruit and Nut Trees and Shrubs of the Virginia Mountain and Piedmont. Virginia Cooperative Extension. https://pubs.ext.vt.edu/ANR/ANR-23/ANR-23NP.html ↵
- Schneider, C. (2014). Encouraging Urban Tree Growth with Organic Soil Amendments. Soil Science Society of America. www.soils.org ↵
- Howard, J. (2017). Anthropogenic Soils. Springer International Publishing. ↵
- Gilman, E. (1997). Trees for Urban and Suburban Landscapes. Delmar Publishers. ↵
- Schneider, C. (2014). Encouraging Urban Tree Growth with Organic Soil Amendments. Soil Science Society of America. www.soils.org ↵
- Vawter, J., et. al.. (2009) A Training Manual for Virginia Tree Stewards Volunteers for the Community Forest (3rd ed.). Virginia Urban Forest Council. http://www.mcleantreesfoundation.org/documents/2020TreeStewardManual.pdf ↵
- Vawter, J., et. al.. (2009) A Training Manual for Virginia Tree Stewards Volunteers for the Community Forest (3rd ed.). Virginia Urban Forest Council. http://www.mcleantreesfoundation.org/documents/2020TreeStewardManual.pdf ↵
- Day, S., Wiseman, E., Dickerson, P., Sarah, B., Harris, R. (2010). Tree Root Ecology in the Urban Environment and Implications for a Sustainable Rhizosphere. Arboriculture and Urban Forestry, 23(5), 193-205. http://wiww.isa-arbor.com. ↵
- Bassuk, N., Curtis, D., Marranca, B.Z., Neal, B. (n.d.) Recommended Urban Trees: Site Assessment and Tree Selection for Stress Tolerance. Urban Horticulture Institute, Department of Horticulture, Cornell University. http://www.hort.cornell.edu ↵
- Scharenbroch, B.C., Smiley, E.T., Kocher, W. (2014). Best Management Practices Soil Management for Urban Trees. International Society of Arboriculture. ↵
- Herms, D.A. (2016, December). Understanding Tree Responses to Abiotic and Biotic Stress Complexes. Arborist News. ↵
- Werner, L.P. (2010, August). Tree Nutrition and Fertilization Practical Considerations (Part II). Arborist News. ↵
- Whitmore, A. (2000). Impact of Livestock on Soil [Address]. Livestock Farming and the Environment: Proceedings of Workshop 4 on Sustainable Animal Production, Hannover, Germany. http://literatur.vti.bund.de/digbib_extern/zi025653.pdf#page=46 ↵
- Scharenbroch, B.C., Smiley, E.T., Kocher, W. (2015, August). Managing Urban Soils That Support Urban Trees (Part two). Arborist News. ↵
- Appleton, B., et.al. (2015). Trees and Shrubs for Acid Soils. Virginia Cooperative Extension Publication 430-027, Virginia Polytechnic Institute and State University. ↵
- Sam Houston University. (n.d.). Soil Properties [PowerPoint]. https://www.shsu.edu/~agr_www/documents/SoilProperties.ppt ↵
- Flemin, G.P., Patterson, K.D., Taverna, K. (2017). The Natural Communities of Virginia: a Classification of Ecological Community Groups and Community Types, Third approximation (Version 3.0). Virginia Department of Conservation and Recreation. http://www.dcr.virginia.gov/natural-heritage/natural-communities/ ↵
- Appleton B, et.al. (2015). Trees and Shrubs That Tolerate Saline Soils and Salt Spray Drift. Virginia Cooperative Extension Publication 430-031, Virginia Polytechnic and State University. https://ext.vt.edu/ ↵
- Werner, L.P. (2010, August). Tree Nutrition and Fertilization Practical Considerations (Part II). Arborist News. ↵
- Virginia Department of Forestry. (2014). Riparian Buffer Zones: Common Plants. www.dcr.virginia.gov/natural-heritage/document/riparian-nat-plants.pd ↵
- Sam Houston University. (n.d.). Soil Properties [PowerPoint]. https://www.shsu.edu/~agr_www/documents/SoilProperties.ppt ↵
- Scheyer, J., Hipple, K. (2005). Urban Soil Primer. Natural Resource Conservation Service. http://soils.usda.gov/use. ↵
- Sam Houston University. (n.d.). Soil Properties [PowerPoint]. https://www.shsu.edu/~agr_www/documents/SoilProperties.ppt ↵
- Whitmore, A. (2000). Impact of Livestock on Soil [Address]. Livestock Farming and the Environment: Proceedings of Workshop 4 on Sustainable Animal Production, Hannover, Germany. http://literatur.vti.bund.de/digbib_extern/zi025653.pdf#page=46 ↵
- Sam Houston University. (n.d.). Soil Properties [PowerPoint]. https://www.shsu.edu/~agr_www/documents/SoilProperties.ppt ↵
- Scharenbroch, B.C., Smiley, E.T., Kocher, W. (2015, August). Managing Urban Soils That Support Urban Trees (Part two). Arborist News. ↵
- Scharenbroch, B.C., Smiley, E.T., Kocher, W. (2015, August). Managing Urban Soils That Support Urban Trees (Part two). Arborist News. ↵
- Sam Houston University. (n.d.). Soil Properties [PowerPoint]. https://www.shsu.edu/~agr_www/documents/SoilProperties.ppt ↵
- Watson, G., Hewit, A., Custic, M., Lo, M. (2014). The Management of Tree Root Systems in Urban and Suburban Settings: A Review of Soil Influence on Root Growth. Arboriculture & Urban Forestry, 40(4), 93-217. www.isa-arbor.com/quizbank/352/193_217_AUFJuly2014.pdf ↵