Chapter 4: Botany of Trees
“A tree trunk the size of a man grows from a blade as thin as a hair” – Lao Tzu
Chapter Contents:
Introduction
What makes a tree different from a tulip? Why do these plants grow so much bigger, stronger and older than all the rest of the Plant Kingdom? This chapter will explore the processes and structures that define woody plants and, within that group, enable trees to stand out (figuratively and literally). Some of the material has to do with chemical interactions at the cellular level, some with organized assemblages of cells such as the vascular systems, and the rest with externally visible functions such as branch structure and root spread. The end result is to see trees as integrated, complex living entities with extraordinary strength, although they face unprecedented challenges in today’s environments. There is so much more to learn, but we can at least start here.
This chapter also serves as a gateway to students’ learning to identify trees for themselves and their ability to look at trees within larger, living contexts.
Learning Objectives
- Understand key plant processes.
- Understand the difference between herbaceous and woody plants.
- Understand what sets trees apart from other woody plants.
- Begin to know how to identify trees.
- Consider the interactions among trees and with their external environments.
REVIEW: VCE Master Gardener Handbook 2015 (9/18 update)
- Chapter 2: Basic Botany
- Chapter 16: Woody Landscape Plants
Key Plant Processes and Definitions
Photosynthesis is defined as the process by which the chlorophyll in green plants use light energy to convert carbon dioxide and water into organic compounds (producing sugars) and water.[1] It is the first and most fundamental step in the support of plant life and by extension all life on the planet. Generally, it takes place during the daytime when the chloroplasts are able to capture light and open the stomata to allow carbon dioxide to enter the leaf. The sugars produced are distributed throughout the plant to keep all the other processes working and the plant growing. Locations which produce more sugars than they use are sources, and those which are net consumers are sinks. Different parts of the plant can function as either one, depending on the season and activity at that location. For example, during new leaf formation, the stored carbohydrates adjacent to the roots’ growing points are the source of provided sugars, and the leaves are the sink; later on in the growing season the situation is reversed.[2] The oscillating rhythm between roots and shoots is a characteristic of the plant plus the seasonal patterns in its environment.
Respiration is the active process by which plants release the energy needed for their living processes, turning sugar and oxygen into energy and carbon dioxide.[3] Chemically, it is the opposite of photosynthesis. Both photosynthesis and respiration require the leaf stomata to open. Through these openings, and some others (e.g., lenticels on twigs/bark), heat from the sun causes water in the stomata to evaporate, thus creating a passive pulling force on the columns of water coming up from the roots. The polarization of water molecules combines with the ionic charges of the xylem to aid in the upward movement of water. (Think of a slinky moving up the stairs instead of down!) Wind blowing across the leaves can enhance evaporation, as does lower relative humidity of the surrounding air. This transpiration process, combined with pressure originating in the roots’ water intake, is essentially the engine which makes the whole plant work. The outer limits of what this engine can accomplish are seen in gravity-defying columns in excess of 300 feet tall in giant redwoods.
Trees, on average, are 80% water (remainder 19% carbohydrates, 1% everything else).[4] As much as 98% of the water which enters the tree exits via transpiration, so it becomes possible to imagine the tremendous amount of movement generated as the water transports dissolved minerals and sugars and acts as a coolant.[5] The water can move as fast as 100 feet an hour in some species at the height of the growing season.[6] All this is in addition to water’s role in the chemical processes already described and its significant role in maintaining turgor, or keeping cells fully plumped up against their rigid walls. The importance of the latter task is very obvious when the water-holding vacuoles within the cell membranes collapse under drought stress, leaving the cell walls holding empty balloon skins. The greater rigidity of woody tissue (compared to annuals and perennials) does give woody plants better structural endurance, but a lot depends upon the appropriateness of the specific plant to the site conditions.
Water is so essential that some plants have developed alternative strategies in response to harsh conditions of heat and/or drought. In one of the two alternatives, the plants have altered the process to take part of the necessary ingredients into one set of photosynthetic cells, then process the reaction in another set, thereby reducing the evaporation potential. The process with which we are most familiar is generally found in climates with reasonable precipitation. It is called C3 because the initial product of the reaction is a three-carbon compound. The two-cell process forms an initial four-carbon compound and is therefore called C4. Wheat, soybeans, zinnias and roses are C3 plants, as are virtually all dicot trees.[7] Sugarcane, sorghum and most grasses are C4 plants. Gardeners in Virginia, especially in warmer regions, are familiar with the choice between cool season grasses such as tall fescue (C3) and warm season ones such as zoysia or bermuda (C4): the latter withstand summer heat and drought far better than the former. The second alternative photosynthetic process takes an even more strenuous step to limit the damage from extreme heat and drought. This group of plants, called CAM after the Crassulaceae family of succulents, opens its stomata at night to make the organic acids which are stored until morning for the remainder of the reaction.[8][9] Cacti, yucca and jade plants are in this category. As a general rule in the contiguous US, the temperate East Coast plants, especially trees, are C3. From Oklahoma/Kansas/Nebraska west, the C4 grassland plants dominate until you get to the desert regions, where CAM plants are more likely. Ecologists are becoming increasingly interested in the possibilities of using more C4 plants to address trends toward harsher, warmer weather patterns and increases in CO2 levels.[10][11]
In addition to water, plants require specific amounts of salt in the soil medium around their roots. If there is too little, it is the equivalent of overwatering, causing cells to rupture. If there is too much, the cells shrivel and may die. The ‘Goldilocks’ amount varies from one type of plant to another but is part of the context that makes a site and plant mutually suitable. Significant changes in soil salinity due to climate change and other environmental factors can be expected to alter the distribution of trees and other plants.
Vascular System. All these wonderful plant processes lead one to expect an appropriately elegant physical structure, and indeed this is so. There are two different ways that trees (and other plants) organize their vascular systems. Monocots, so called because their seeds each contain just one cotyledon (or seed leaf) leaf, have vascular bundles throughout their stems. This means that monocots do not have branches nor woody root systems. Dicots, with two cotyledons inside each seed, place their vascular bundles in a ring near the outside of the trunk. Newer, genetically based research has further divided the dicots based on their evolution (discussed in Chapter Five) but the distinctions are of little practical impact to this chapter’s discussion of tree structure and function. The only monocot trees are palms, so EMG Tree Stewards in Virginia will spend most of their time with dicot trees.
Water, minerals and hormones are moved up the plant by xylem tissue, tube-shaped cells which conduct waters and dissolved minerals from the roots through the trunk to the branches, leaves, flowers and fruits. Dicots, and some monocots, have both larger xylem vessels and smaller tracheid tubes, while the dicot conifers have only tracheids.
The second component of plant stems and roots is the phloem tissue, which moves sugars up, down and around. Its cells are minimal in structure and aided by companion cells to complete all its functions. In dicots, the xylem is on the inside (nearest the heart) and the phloem is on the outside (nearest the bark).
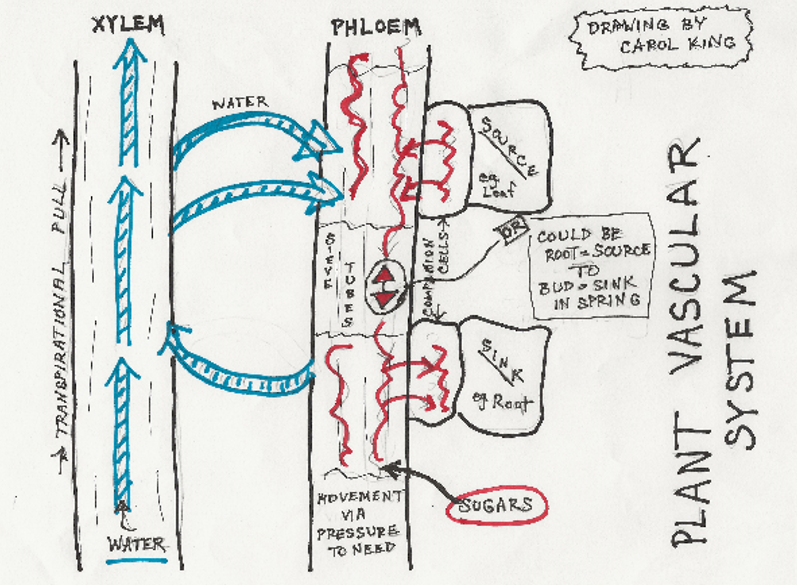
The genie in the system is the meristematic tissue. This is found between the xylem and phloem and allows new cells to turn into either one, depending on the location and need. The annual growth rings visible in Figure 4-2 show the difference between early growth each year (tending to have larger vessels) and the later part of the year. In good growth years, these rings are often larger than in harsher years, a pattern which has been very valuable to researchers in many fields. The picture is actually a branch rather than a trunk, but the principle is the same.
It is worth noting that dicot trees have both apical meristem and lateral (vascular) meristem, meaning that a tree can produce new cells capable of different roles both in the cambium and in the ends of the branches and roots. This is one of the features that distinguishes them from other plant types.
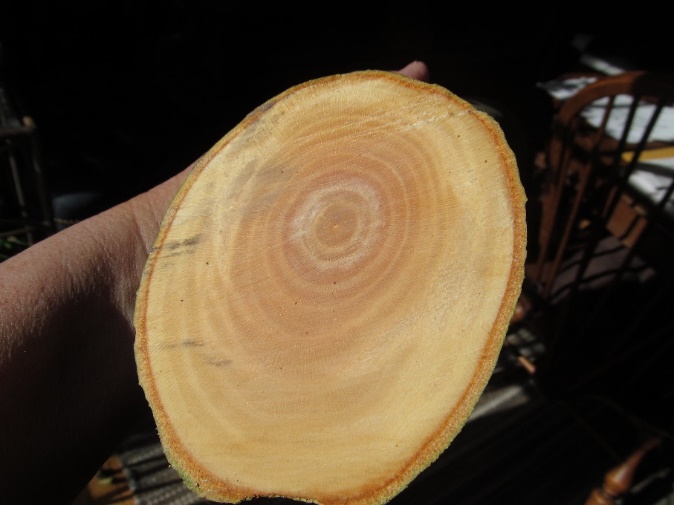
In buds and the root zone of elongation, the meristematic tissue is the beginning of all the plant cells. The next, larger complex of meristematic tissue is the cambium, which is constantly renewing both phloem (outside of the cambium) and xylem (inside the cambium), in harmony with the plant’s seasonal annual cycle. In dicots the system of vascular tissue is found near the outer edge. Since the new growth in the spring is larger than that in the fall, the annual production of new vascular rings is visible, with that year’s growing condition reflected in the size of the ring. In monocots, the vascular tissue is scattered generally throughout the stem, and the phloem activity causes the vascular bundles to expand inside the trunk, gradually pushing the whole structure to a larger dimension.
Rays are a significant part of the woody picture for dicots, radiating from the center to the exterior like slicing a pie. Within the live tissue of the trunk, they are living cells which can store and transport food, and also help with the sealing off of damaged tissue. In the tree as a whole, they function as structural ties to the center of the trunk (tapering from the outside) and thus help stiffen the trunk and make it more stable.[12]
The few monocots that achieve the size and function of trees, such as palms and bamboo, have xylem and phloem joined in bundles rather than rings. Such arrangements can produce notable flexibility, but these plants also rely on fibrous roots systems for additional stability.
Plant Hormones and Tropisms. Plant hormones are chemicals which are produced in small quantities in specialized cells in one part of the plant and transported elsewhere to trigger responses in plant processes. These are also called plant growth regulators (or PGR), since that is what they do. They are fundamental to the functioning of all plants, but some of their effects are especially visible in the life of trees. They control the development of trunk and branch structure and facilitate root growth and leaf abscission, among many other functions. Usually, the plant response depends not so much on the quantity of the chemical as on the interaction with other plant hormones. The subject is one of much study and considerable complexity. The following are the most significant ones for the purposes of Tree Stewards’ knowledge, but it is not an exhaustive list.
Auxin was the first plant hormone identified by scientists. The major auxin in plants is indoleactic acid or IAA. It is primarily responsible for apical dominance, using cell elongation to promote the growth of the primary stem and branching structure as well as the development of new roots by translocation. It is produced mostly in the ends of the branches and trunks, then travels through the cells to where it is needed. In conjunction with cytokinins (see below), they also suppress lateral buds in the branch tissue below the tips, ensuring that water and nutrients are directed preferentially to the apical tissues. When apical dominance is broken, by cutting off the ends of trunks and branches, the mix of hormones is disturbed and the plant adapts with a new growth strategy. The implications for pruning will be seen in Chapter Nine.
Cytokinins stimulate cell division and differentiation, among other effects. They are produced in actively growing tissues, especially roots and fruits, whence they move to target locations in the xylem sap. As said above, the interaction of cytokinins and auxins keeps cells growing, dividing and differentiating in a healthy pattern.
Gibberellins stimulate stem and leaf growth by enhancing cell elongation and division. When garden vegetables go to flower and then bolt, this is an excess of gibberellin at work. They are also significant in signaling a seed embryo to break dormancy. They are largely produced in young roots and leaves. Gibberellins combined with auxins are also necessary for many plants to develop fruit. Gibberellins are part of a group of chemicals called Plant Growth Regulators (PGR) which can play a valuable role in urban tree maintenance by managing tree growth rates so as to reduce pruning needs and help the trees handle stress.
Abscissic Acid was originally, and erroneously, thought to be involved in bud dormancy and leaf abscission (leaf drop) in deciduous trees, hence the name. Actually, this hormone slows growth, which helps keep dormant seeds safe from premature germination and also helps the plant deal with drought by closing the leaf stomata guard cells.
Ethylene is a plant hormone which is also a gas. It is well known for its importance in the ripening of fruit. When you put part-ripe peaches in a paper bag, you are using ethylene gas to finish the ripening process. This hormone is produced in response to stress, during fruit ripening, as a part of programmed tissue death (leaf drop), and as a part of the natural death cycle of plants.
Tropisms: This is a term from the Greek (tropos = turn) for a plant response which involves organs deviating from a straight growth pattern in response to some kind of stimulus. The most famous of these is phototropism, which is the bending of plant stems toward sunlight or equivalent light source. This happens because the cells on the shady side of the stem elongate more than on the sunny side due to the combination of hormonal action. Gravitropism or Geotropism is the other effect of significance to Tree Stewards: this is how the stem of a plant knows to grow up and the root to grow down. It is not purely gravity, but also hormonal effect upon gravity-influenced cells. When you tie down an upright grapevine or rose cane on a horizontal support, you are tapping into both the gravitropism (which way is up?) and apical dominance (where are we going?) of the plant.
Particulars of Woody Plants versus Herbaceous
Woody plants are defined as perennials which retain a portion of their structure above ground all year long. If the plant is deciduous, it will still keep a live stem or trunk through winter, possibly in a dormant state. For a woody plant to survive external threats over years or even centuries, it must create some kind of a protective layer and wound response strategies. In order to thrive, the plant must continue to grow, adding bulk during the active season. Both of these processes mean additional weight, so the plant must also have a plan for supporting itself against the forces of gravity, wind, and water. The really delicate part of the equation is that the plant must also produce the energy to perform its principal function (from its perspective) of reproduction: cones, flowers, fruits and seeds all take considerable effort to achieve.
So what is wood, anyway? Technically, it is secondary xylem, meaning the tissue in rings (dicots) or bundles (monocots) from past seasons’ growth. The tissue between the xylem tubes gradually thickens to wood in maturing trees and shrubs. Thus we get the solid, mostly dead heartwood giving dicots their solidity and strength, and also the diffuse pattern of tracheids and surrounding older vascular tissue which leads to the lightweight, flexible nature of monocots such as palm trees and bamboo.
The key materials in making wood are cellulose and lignin. Cellulose is a structural polysaccharide, a polymer made of glucose. It is the most abundant organic compound on Earth.[13] It is related to, but not the same as starch, which is a form of glucose essential to other parts of plant functioning. The particular structure of cellulose makes it an ideal material for cell walls: soft yet tough, it is also resistant to tension forces. Lignin is a stiff polymer which is key to the function of xylem tubes (think drinking straws). It anchors cellulose fibers and cements them together. In contrast to cellulose, it resists compression. It is the second most abundant natural polymer in the world.2 The combination of the two is what makes it possible for woody plants to ascend to heights as they grow in girth.
Wood grain is the pattern or orientation of the growth of the secondary xylem and is generally spoken of in connection with lumber production, woodworking, and splitting for firewood. In these contexts, the xylem tubes are often referred to as fibers. Most tree species have a typical pattern, but this, and other wood properties, can be influenced by location and growth condition.[14]
The simplest pattern is straight grain, when the fibers run parallel with the vertical axis of the tree or log. diagonal grain seen in the sawn wood is the same thing from the tree’s perspective, but with the saw at an angle to the log’s vertical axis. Trees with knots or other internal obstacles that divert the fibers produce an irregular grain, which can be attractive but tricky to work with. Wood with a pattern of small direction changes has a wavy grain (apparently more a genetic feature), while trees of many species may develop spiral grain (fibers winding around the tree either left- or right-handed) in response to adverse growing conditions involving sustained unequal stress.[15][16]
Finally, a number of species are fairly famous for tending to have interlocked grain, which means the fibers grow in one direction one year, then reverse direction the next, repeatedly. Among these are sycamore (Platanus occidentalis), blackgum (Nyssa sylvatica), sweetgum (Liquidambar styraciflua), and many elms (Ulmus spp).[17][18][19] Anyone who has ever tried to split any of them for firewood is only too familiar with the difficulties presented by these odd trees. But the pattern seems to be deliberate, hence we may surmise that the trees have a purpose. The wood from such trees can be used for applications which need a resistance to torque, for example. Perhaps the tree is aiming for a similar flexibility itself?
Another key element in the nature of wood is density. Greater density comes from a larger proportion of xylem and tracheid cells with thick walls and less void space.[20] Factors behind different densities are both genetic and environmental. The density of any particular species and tree is important for the harvested wood’s commercial value and best use. Considering the living tree, greater density means more weight: is the tree then stronger and better able to withstand environmental stresses? The Bristlecone Pine is an interesting case in point. They grow slowly and live fantastically long, up to 5,000 years, in an environment with high drought and wind stress; and their wood is indeed very dense.[21] Yet there are environments where tree wood tends not to be dense: typically more favorable growing environments or moist tropical situations. Trees with rapid growth habits (weeping willows, e.g.) generally have less dense wood so they can compete early on, but they are not usually long-lived.
Particulars of Trees versus Other Woodies
Woody plants include not just trees but shrubs, vines and ground covers. Vines and ground covers clearly have different survival strategies and don’t seem to have potential to become trees. Shrubs are another matter, and indeed some woody species can be either trees or shrubs depending upon the variety, climate zone, and site circumstances.
The definition of a tree is a woody perennial plant, typically having a single stem or trunk, growing to a considerable height and having lateral branches at some distance from the ground. In contrast to a vine, it has a self-supporting structure. The Virginia Big Trees data base determines a tree by the minimum measurements to qualify for inclusion: 13 feet in height and trunk circumference of 9.5 inches, measured at 4.5 feet above the ground (DBH, or Diameter at Breast Height). Any species with mature measurements below these minimums can be considered a shrub. While this is not a definitive formula, it is a useful way to differentiate trees and shrubs. It is worth noting that in the horticultural world, some normally shrubby plants as wax myrtles and viburnums can be trained to a tree form with single stems and clearly defined crowns.
Roots
Roots are the foundation of a tree, literally and functionally. They anchor the plant (no small feat of engineering); bring in water and nutrients for the entire structure; and store food to allow the tree to survive harsh times such as winter. Roots are also a location for hormone synthesis and soil symbiosis. Although roots tend to be among the last parts of a tree most people think about, they are routinely the source of many problems in the urban landscape, mostly in the form of damage to infrastructure (sidewalks and building foundations) and from a safety aspect (trip hazards). When a tree’s roots are compromised by poor soil conditions, excessive water or salts, or mechanical injury (just a short list), then the upper part of the tree will eventually pay the price.
The first thing a tree seed does upon germinating is to send out an absorbing root with lots of root hairs behind a little root cap. Keep in mind that the activity in the absorbing roots is paramount to the tree’s overall success, and yet these roots can be measured in millimeters and require a microscope to see. These single-cell root hairs are the primary intake mechanism for water and minerals throughout the life of the tree: they are found in the zone behind the root cap and before the root starts to become woody. In terms of function, they are the primary source of water and minerals from the soil. As the root lengthens and enlarges, the root hair zone remains just behind the newest, small tip. Thus the absorption roots are found at the outskirts of the main roots, especially in the first several inches to foot of the soils surface, where water and nutrients are most available.
Trees and Sidewalks
When selecting street trees for sites that are largely paved, major consideration should be given to the expected root system depth as well as the tree’s known tolerance for hypoxic conditions. Caution is warranted with most if not all maples, many ashes, and the American elm. Willows, poplars and silver maples are not just shallow rooted but can be destructively invasive near water or sewer pipes.[22][23]
In dicots, the first woody root to develop is generally a tap root, which extends downward to anchor the new tree. Most trees do not continue the tap root once the Lateral Roots begin to grow. In some species, this appears to be genetic, for example maples.[24] In others, this can be a response to oxygen, water and mineral levels in the soil horizons due to water saturation, extreme compaction or soil chemical concentrations.[25] In nurseries, tree seedlings usually have their tap roots cut in order to keep their root balls at a manageable size. There is some art to doing this properly: current research has looked at the better effect of air pruning young seedlings (containers with holes in the sides or soft fabric containers, for example) instead of mechanically chopping off the root end.[26]
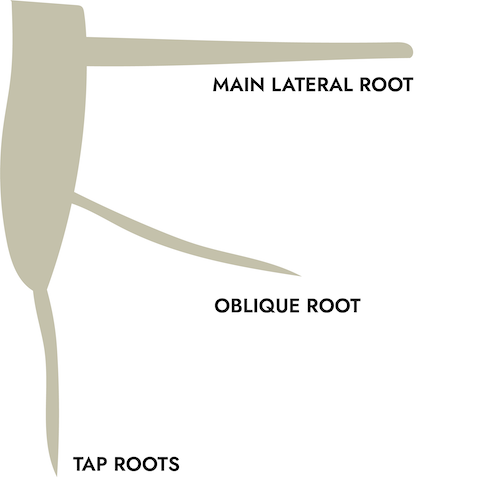
Lateral roots grow at right angles to the trunk and ideally extend straight outwards, as seen in the photograph below by Dr. Ed Gilman of a good quality young root structure. The lateral roots provide the greatest stability to the tree and extend the greatest distance from the trunk: depending on the species, soil and site conditions, as much as 3 times the canopy spread.[27] In trying to estimate the full root zone of a mature tree, one should look at the trunk diameter as well as the conditions under which the tree has grown.
Sinker roots may be sent down from laterals for additional anchoring. There may also be oblique roots which originate in the root flare and create additional stability by growing at an angle downwards. If one visualizes shallow root structures as the foot of a wine glass on a dinner plate, then those with oblique roots are more like the foot of the wine glass on a plate set upon a shallow bowl.[28]
The trunk flare or root collar is the interface between the trunk and root system. This area functions as a shock absorber because of the annual growth rings that are up to twice the size of the annual growth rings further up the trunk. Since the root collar is supposed to be right at ground level (roots below and trunk above, with the flare visible), it is also an area of great importance to a tree’s survival. The unfortunate practices of planting too deeply, piling mulch up around the trunk and using weed-eaters right around the base make tree failure due to trunk girdling all too common in urban settings.
Stem girdling roots are laterals which have chosen to grow too close to the trunk, or may be adventitious growths from a poorly organized root system. They may also be a legacy from container-grown problems with small circling roots which were left uncorrected. As the girdling root increases in diameter, it compresses the phloem and disrupts water flow in the xylem by breaking the cambium layer.
Generally, though, most tree roots are in the top 3 to 6 feet of soil, closer to 3 feet in the human environment. There are trees which do put down deep tap roots due to genetics, plus soil and water. Most oaks, walnuts and hickories fall in this category. Also, trees which survive in watery environments (bald cypress (Taxodium distichum), for example) may have to put down fairly deep roots. The bald cypress uniquely has roots which protrude above the water or soil, probably to access oxygen.
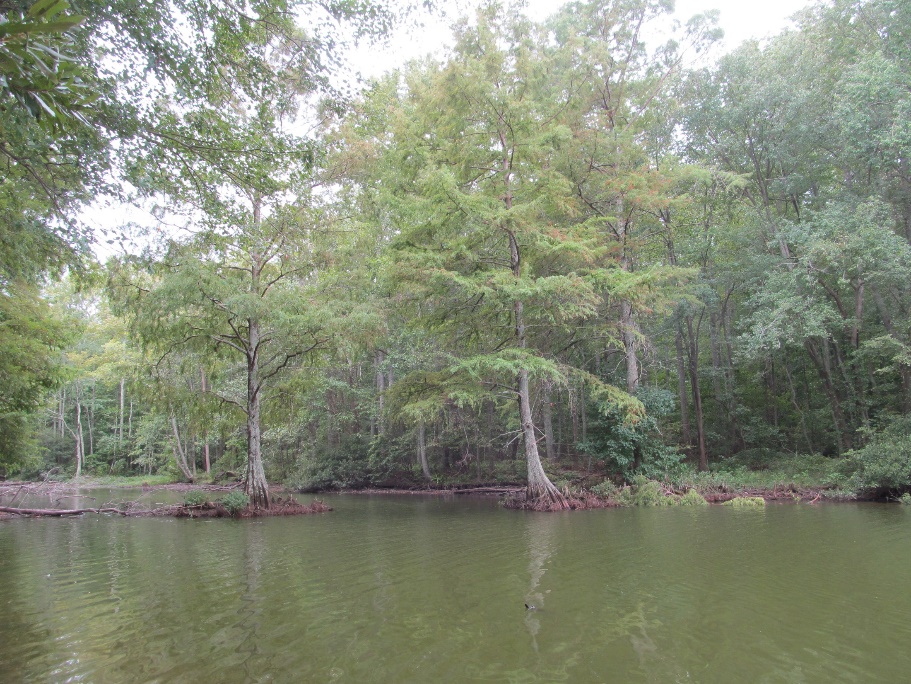
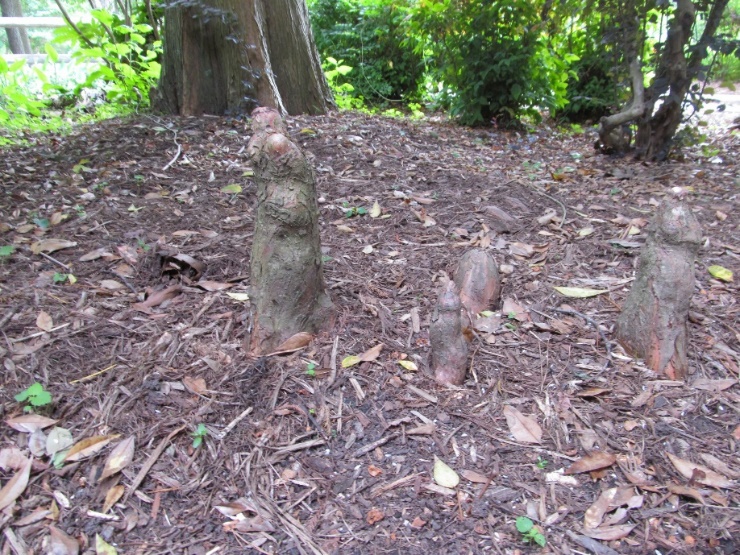
The root plate is the shallow complex of large roots, small roots and soil that adheres around the base of the trunk. It is usually three to five times the diameter of trunk. It is largely responsible for holding the trunk upright.[29][30] The root plate is associated with buttress roots, which shore up the trunk by extending the root flare without making it dense, as seen in the northern red oak at right below. Gothic cathedrals use this principle to spread the load of the roof across a wider span, as also with athletic stadiums.
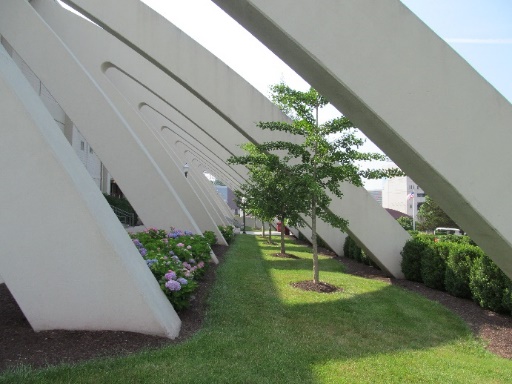
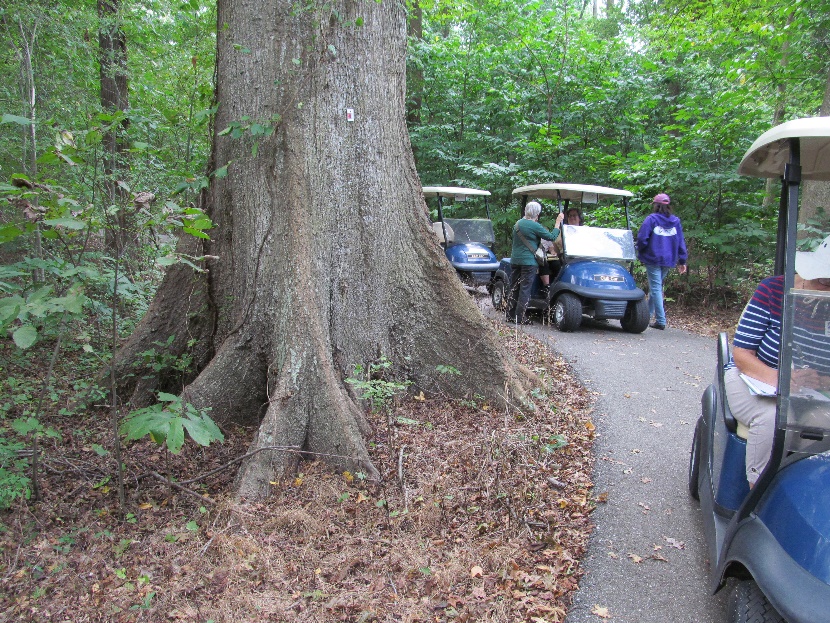
Buttress roots act as stabilizer bars for the tree. They extend through the root plate (6 to 8 feet past the trunk flare, for many mature trees), then the roots decrease drastically in diameter (from 8 to 10 inches to 2 to 4 inches). This point of decrease is known as the zone of rapid taper. Root systems normally heave or fail at this zone. When trees fall over, the root plate is then visible: a failure of roots but also of the soil. This can happen from swampy conditions in a forest (Figure 4-8) or from wind stress when the roots are not stable (Figure 4-9).
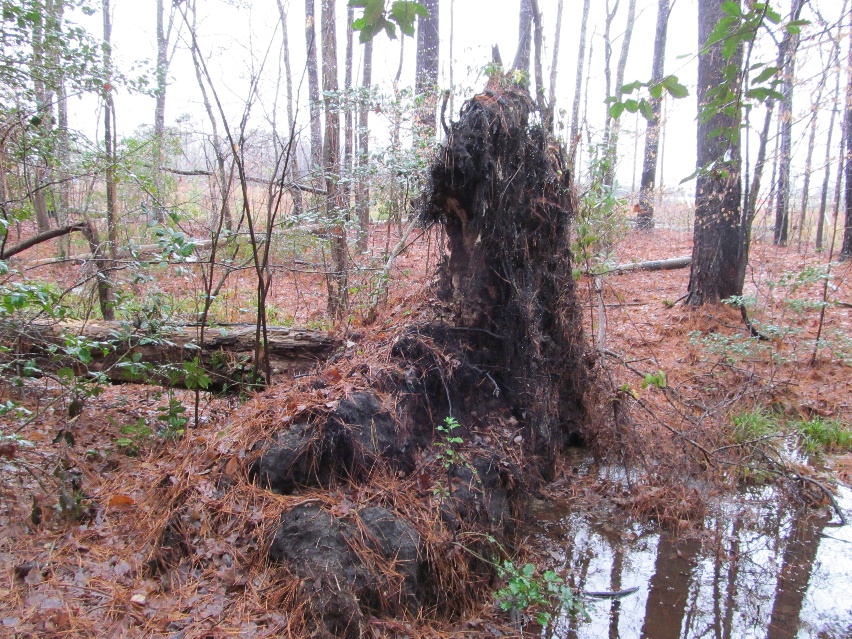
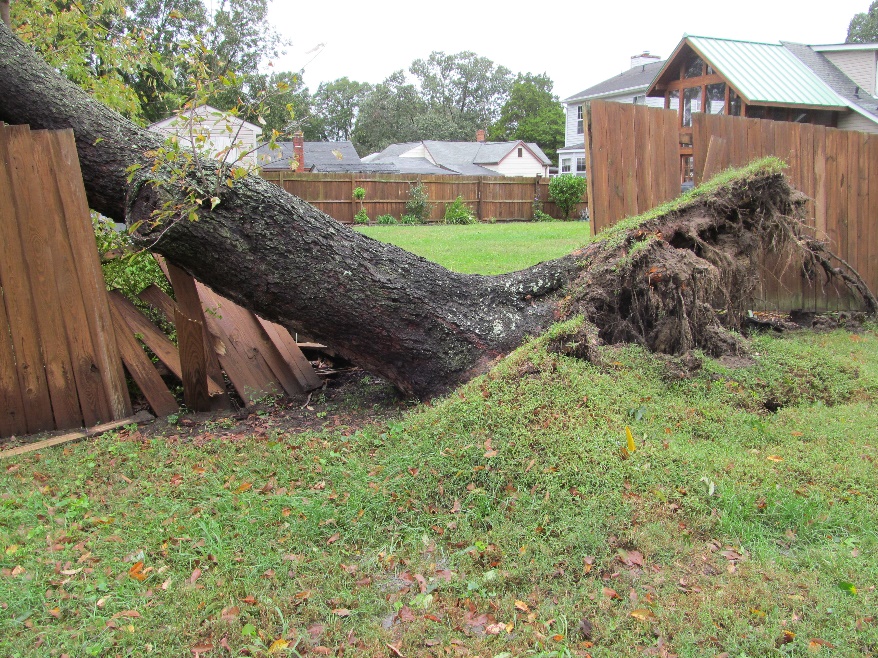
One more type of root is worth watching for: Adventitious Roots may form when the trunk is buried in soil or mulch. They are less well attached than the roots which develop naturally in the course of the tree’s growth, and they will keep some water and nutrients from the lateral roots which are in root system proper.
Here is an interesting question: Do dicot roots have rings? The answer is yes, which makes sense if one considers that the vascular system runs the length and breadth of the tree. This means the water which enters the xylem in the roots must connect to that which feeds the smallest leaf, and similarly for the phloem. However, the wood of the roots is not subject to the same stresses as that above ground and does not develop as robustly. They have more thin-walled live cells than do trunks, and they do not have pith. They are thus less cold hardy than woody tissue aboveground. Also, roots are more likely to encounter obstacles (rocks, sidewalks, patches of nutrient or water discontinuity) and have to grow around them.
Regarding monocots, the vascular system has to include the roots in some mechanically consistent way, which means that the many distributed vascular bundles can only form a lot of small root connections from the base of the trunk, without a root flare or woody branching. This is called a root-stem transition zone. Such trees have an entirely fibrous root system which operates at a shallow level: but the trunks are lighter and more flexible because they do not have the weight of the dicot annual ring-layering.[31][32][33][34]
Identification by Roots: Roots are not usually a help to the EMG Tree Steward trying to identify a species of tree. First, they are often not easily visible. However, a shallow rooting pattern, especially in good soil, may be somewhat of a clue. Plus, there may be some information to be gleaned: color (yellow for white mulberry), or odor (root beer for sassafras), and odd growth (bald cypress knees, for example).
Any current discussion of roots is not complete without the rhizosphere. Definition: the region of soil that surrounds, and is affected by, plant roots.[35] It includes not only all the important soil constituents (see Chapter Six) but also the activity of the small fungi, known as mycorrhizae which live symbiotically with the tree roots. These fungi combine with the small absorption roots, sending hypha into and between the root cells to enhance the roots’ intake of water and minerals. In return, the fungi get some of the food the leaves have made, including sugars, as well as keeping some of their soil harvest for themselves. Root turnover and root exudates play an important role in carbon cycling, soil chemistry and food web nourishment.
While we are in the Rhizosphere, this is a good time discuss an interesting function by which plants seek to obtain an advantage for their survival and reproduction. Allelopathy “involves a plant’s secretion of biochemical materials into the environment to inhibit germination or growth of surrounding vegetation.” The Black Walnut (Juglans nigra) is the best known example, observed back even to Roman times. The chemical juglone is found in all parts of the black walnut tree, but its effect is mainly from roots and decomposing leaves, killing off any of the selected plants which cannot tolerate the chemical. There are other trees which produce allelopathic chemicals to a lesser degree, including hackberries (Celtis spp), sycamore (Platanus occidentalis) and black cherry (Prunus serotina).[36]
Trunks and Branches
Trunk and Branches are the next and probably most studied element of dicot trees. This is where we see wounds, pest damage, and overall tree failure when the structure is not up to the load being placed upon it. This also where we see the bark providing protection against temperature extremes and invasion by pests.
Compartmentalization is a process by which a dicot tree sets boundaries around injured or decayed wood so as to protect healthy remaining wood from disease-causing organisms. The most broadly accepted model (though not the only one) is modified from that proposed in the 1980s by Dr. Alex Shigo: Compartmentalization of Damage in Trees or CODIT. It is complex and involves both chemical and physical steps. When the tree is wounded, air rushes into the vascular system, followed by bacteria and fungi. If the wound is caused by branch removal, severing part of the vascular system, then sudden auxin loss may also be a factor.[37][38]
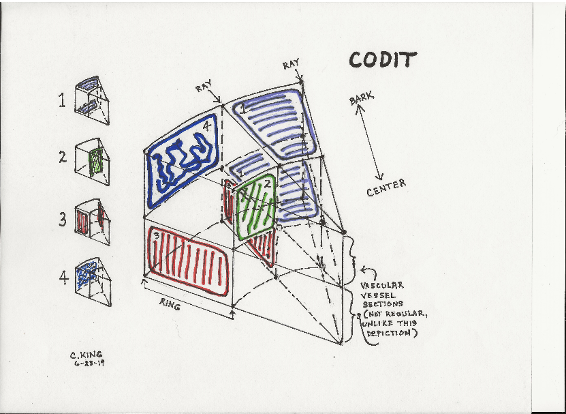
In the CODIT model, the tree then uses stored energy (at a cost to its other functions) to (1) plug the vessels above and below the damage, (2) cause the live cells in the ring lying between the injury and the center of the trunk to produce chemicals toxic to fungi, (3) stiffen the rays on either side of the wound with a wax-like chemical and, finally, (4) form a barrier zone from the cambium along the outermost growth ring at the time of the wound. This last barrier will remain in that ring but can spread around in the ring over time. The four walls are listed in increasing order of strength, hence it is easier for disease to spread vertically than to break through into the interior, across the rays or through the outer wall.
- Wall 1: stops upward movement of decay. Weakest Wall.
- Wall 2: stops inward movement of decay.
- Wall 3: stops lateral spread of decay.
- Wall 4: new growth ring. Strongest wall chemically but weakest structurally because Walls 1 to 3 usually result in a pocket of decay.
Not all trees can succeed in erecting all four walls. Trees under stress have less energy to spare, and some wounds are too severe to fully enclose. The process walls off any sugars stored in the wounded zone, and the tree also loses a portion of its productive tissue forever. Also, Wall 4 is chemically strong but structurally brittle, so may subsequently crack if stressed in a way the tree isn’t used to.[39]
Trees vary in their ability to compartmentalize, both individually based on vitality and as species. This should be one of the criteria in selecting trees for urban street sites or high-traffic areas.
Reaction wood. The main component of dicot trunks and branches is the wood formed by the annual rings, which are influenced by genetics, weather, and the productivity of the tree’s internal processes (water, food, and so on). Trees are able to react to tropisms and constant wind load by forming reaction wood. Reaction wood is an increase in cellulose or lignin between annual growth rings on one side of the branch or trunk. There are two types of reaction wood. The function of Tension wood is to ‘pull up’ the branch or tug on the trunk. This tissue has a larger component of cellulose (which resists tension but doesn’t handle compression well). Compression wood has a complementary role. Its function is to ‘push up’ the branch or trunk. It has a larger portion of lignin, which resists compression but doesn’t handle tension well.[40] Figure 4-11 shows compression on the underside of a pine branch. The combined picture of a stable tree with basic wood plus compression and tension wood helps us understand why trees can survive many years in a tough spot, yet fail when a sudden storm brings pressure loads from an unexpected direction.
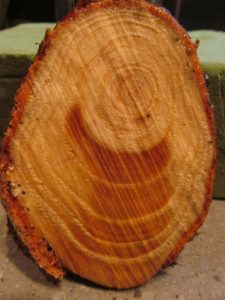
The bark is one of the three preset defense barriers dicot trees have developed to aid in longevity. The others are: pith, which is central nonvascular tissue inside of the vascular ring system, and the branch attachment system, composed of the branch bark ridge, branch collar, branch protection zones and other aspects of the tissue complex where the branch joins the trunk. The former performs various specialized functions while the latter supports the branch and tries to keep branch infection from reaching the trunk.[41][42]
The trunk is the main vertical weight-bearing mechanism for the entire tree. From an engineering perspective, a single straight shaft would be more efficient, but the tree would probably not be able to find room for enough leaves to support itself. So the branching function, and particularly the way the branches attach to the trunk, are critical to the development of trees as we know them (again, dicots). The attachment must provide a ready flow of the vascular system yet also support the ever-increasing weight of the branch. The patterns of xylem growth show layers growing around the attachment point and stiffening into wood as the trunk and branch.
The diagrams in Figure 4-12 show how the vascular system goes up the trunk into the lower side of the branch. Then tubes on the upper side of the branch bend round and continue on up the trunk. At the junctures of trunk and branch the layers from each alternate as the tree grows. The right diagram shows one of the disadvantages of a narrow branch angle, trapping wood and bark into a divisive wedge which can lead to problems later.
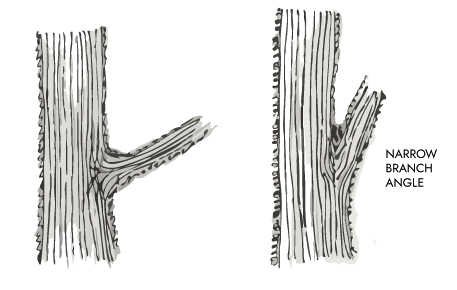
There will be a branch collar at the base of the branch where the wood makes an abrupt turn downward and forms overlapping layers with the trunk xylem. If the branch angle is proper (as at left above) this will make a zone of especially strong chemical and physical support. In order for this process to work, the branch must be smaller in diameter than the trunk; if they are the same size, neither one can encompass the other’s xylem, so the union will not work well. So, not only are co-dominant stems mechanically weak, but biologically weak as well. The relative size of branch and trunk is called aspect ratio. 1:3 is considered ideal for strength. Anything larger (1:2 or 1:1) is not as stable an attachment.[43][44]
There is one more consideration in branch health: the timing of the formation of the branch. If a branch develops at a natural time in the tree’s life cycle, the start of the attachment point will be deep within the trunk when both have achieved proper size. By contrast, a later shoot that arises from a pruning or other cut will have a much shallower level of attachment and be inherently weaker. Water sprouts are a case in point due to the fact that that are vertical, fast growers and usually form at perpendicular angles to the parent branch. Storms, pests, and physical injuries are some of the causes of sprouts.
Figures 4-13 and 4-14 show good development of an original branch in a red maple and an older red maple with a proper branch below some new ones which have grown from the cambium around a cut-off branch above it. The newer branches will be weakly attached and fragile until the tree grows around that point.
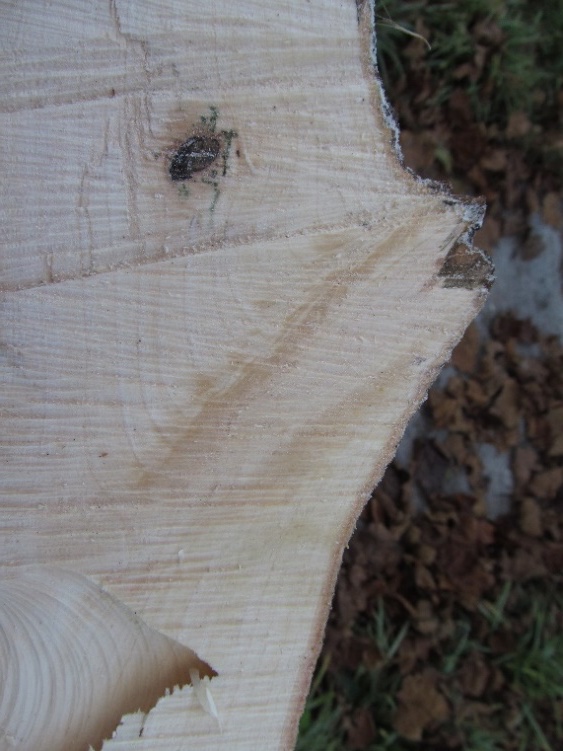
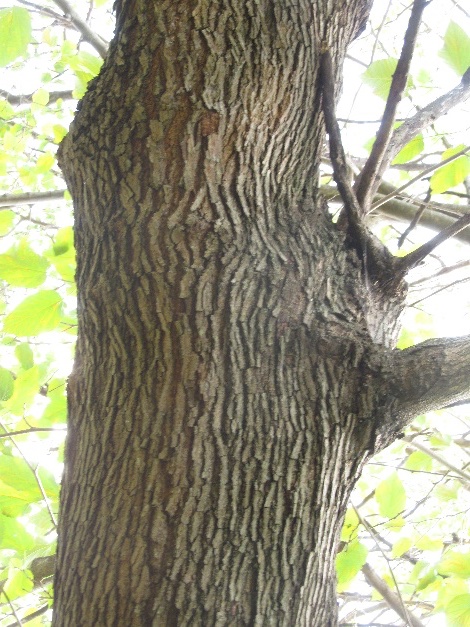
Crown Form is the essential shape of the tree. The words crown and canopy are often used interchangeably, but there is a difference. Crown is the totality of the leaves and branches measured from the lowest branch to the topmost growth. Canopy is the mass of leaves and small twigs within the crown.[45] Excurrent crowns feature a dominant central leader with many horizontal branches. Decurrent crowns have several codominant branches, leading to a rounded or flat-topped appearance. An individual tree’s genetics, influenced by its environment, will often produce characteristic patterns identifiable from a distance.
Branch Structure is an important component of tree strength and health. We have seen that a wider angle of attachment is better mechanically, plus it can also give more leaves a chance to get sunlight. The genetic branching pattern is one key consideration in tree selection, particularly if it is to be a lone landscape tree. The environment in which the tree is growing may require extra attention to the balance between branches and trunk: this is particularly true for trees in the open (wider, lower branches) and those in crowded or highly trafficked areas (narrower branches and trunks cleared higher).
Hollow trunks in dicots are caused by some kind of disease or wound, but the tree may be able to survive nonetheless. Assuming the infection has been contained, the tree’s vascular system is on the outside so can function perfectly well. The tree will have less structural bulk and weight, but if the cylinder wall is intact, it is mechanically sound. Dr. Gilman advises that “a tree trunk can loose [sic] up to about 70% of its wood cross-sectional area in the center and still retain about two-thirds of its strength provided there are no openings in the trunk.”[46]Some trees are also prone to having hollow trunks, such as older forest-grown basswoods (Tilia americana).[47] Turning briefly to the monocots, the structure lends itself to creating a hollow, flexible cylinder, as in bamboo.
Identifying Trees by Trunks: There are three aspects to consider: structural patterns, bark and twigs.
Structural patterns have been discussed earlier in this chapter, but it is worth thinking about your first impression when you look at the tree. Is the trunk straight? How tall compared to its girth? Is there a tendency to multiple leaders? Are the branch angles narrow, more level, or even drooping? You might even observe a tendency to sucker, which could be a clue.
Bark is often hard to identify, but certain trees have very distinctive textures and colors: this can even be the principal ID feature, as in American persimmon (Diospyros virginiana) whose dark, blocky bark can be seen as almost charcoal-like; the smooth blue-gray bark of the American beech (Fagus grandifolia) pictured in Chapter Three; and the corky ‘warts’ which distinguish the hackberries (Celtis spp).
Twigs are specifically the last year’s growth in a branch. Comparing twigs with growth from previous years can help estimate a young tree’s vigor. In addition, depending on the species, twigs and young branches may have special ID features. These are often of greatest importance in the winter.
As an aid to understanding the parts of a winter twig, Fig 4-15 may be helpful. Not all twigs have all the features shown, and there is considerable variety in the actual appearance of each feature among different species.
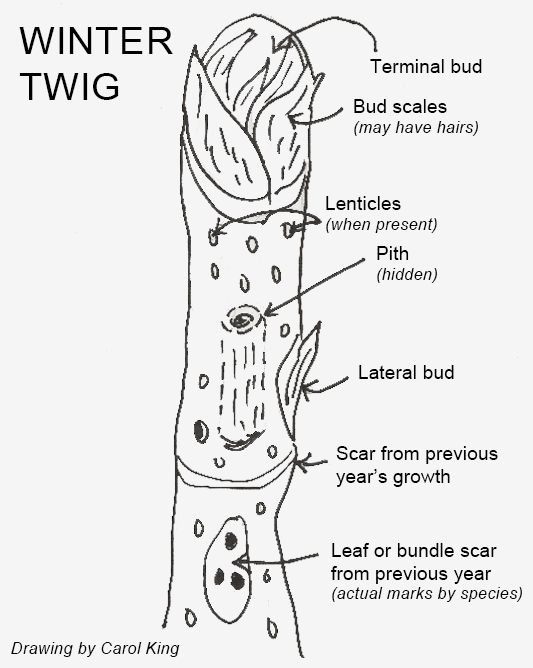
Twig color and relative size are the first things to notice, then the pattern of buds. Buds may be located at the actual end of the twig (end bud true) or offset from the very end of the twig (end bud false). They may have scales (seen with a hand lens). Tthe shape may be distinctive: beech buds (Fagus grandifolia) are long and narrow, like a paint brush. Tulip poplar buds (Liriodenron tulipifera) look like a duck’s bill, and magnolia buds are typically fuzzy. Or their arrangement may be informative: oaks generally have clustered end buds.
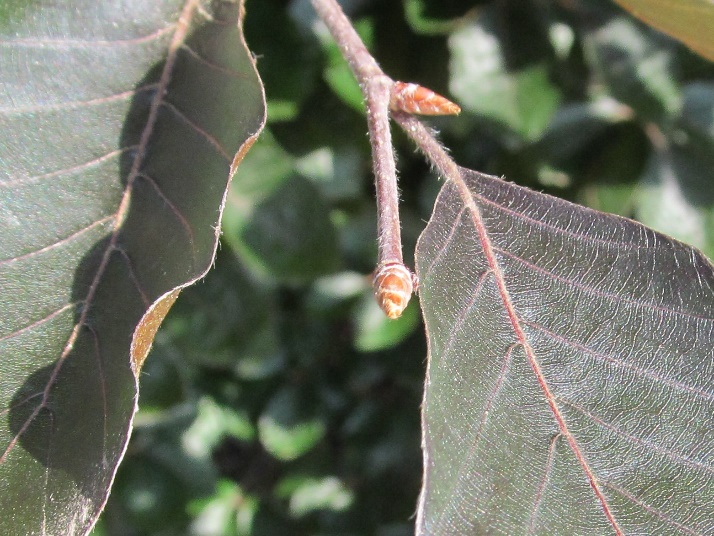
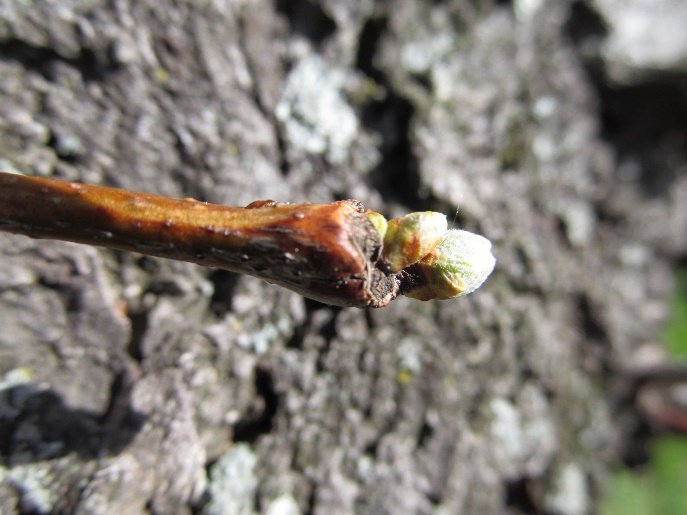
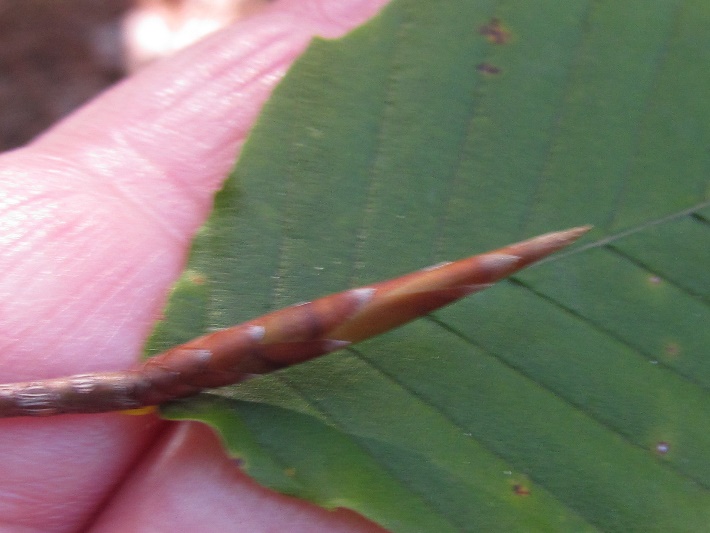
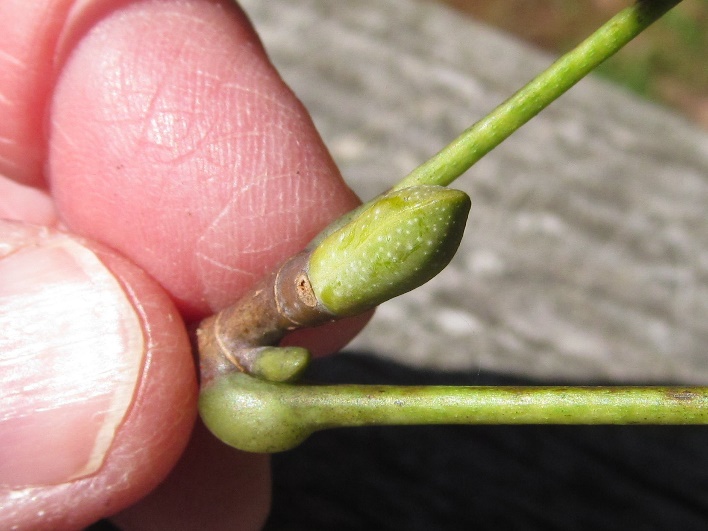
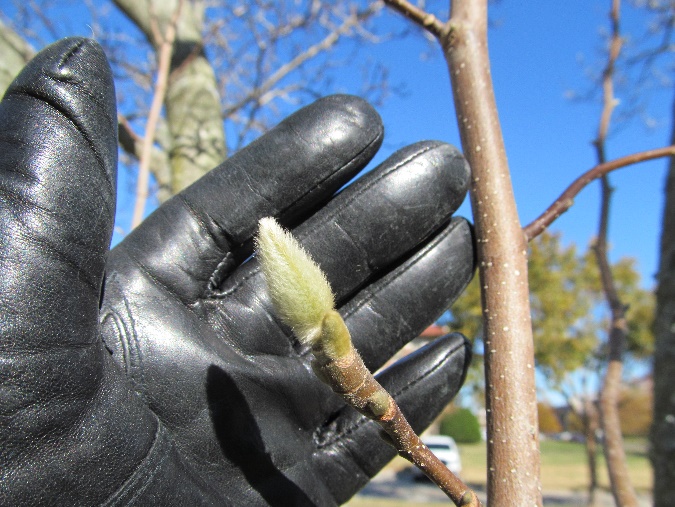
Two further twig features may also be useful in winter ID.
Leaf or bundle scars are what is left on the twig after the leaves have fallen. The pattern of the vascular system connections to those leaves is highly distinctive and can tell, for example, the difference between the shield shape of a green ash (Fraxinus pennsylvanica) and the smiley face of a white ash (F. americana).
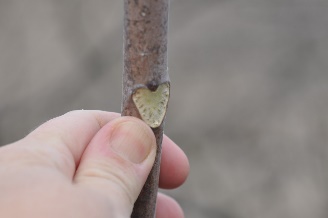
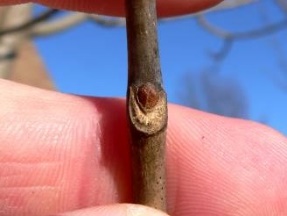
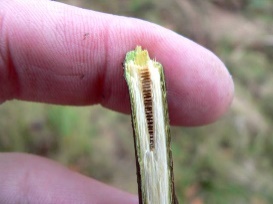
Pith is the center of the twig and is one of the last ID features consulted, as most are pretty much straight cylinders of woody tissue. However, for those trees with some distinctive pith, this may be worth checking. One example is the black walnut (Juglans nigra), which has an unusual dark brown, chambered pith. An unusual feature found in some trees can be helpful: wings. These may be distinctive corky growth in the twigs and young branches of the winged elm (Ulmus alata) and juvenile sweetgums (Liquidambar styraciflua). Note that there are other sorts of wings found on trees and shrubs: this word also describes the green leafy tissue along racemes of the winged sumac (Rhus copallina), for example.
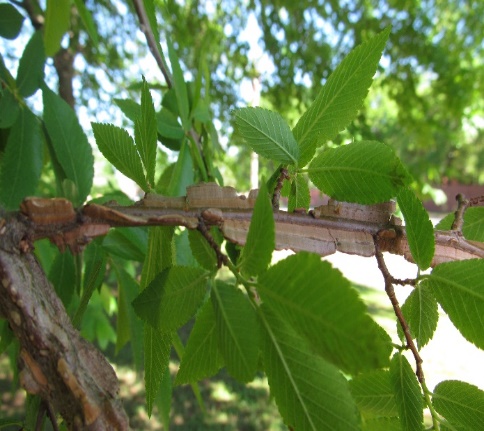
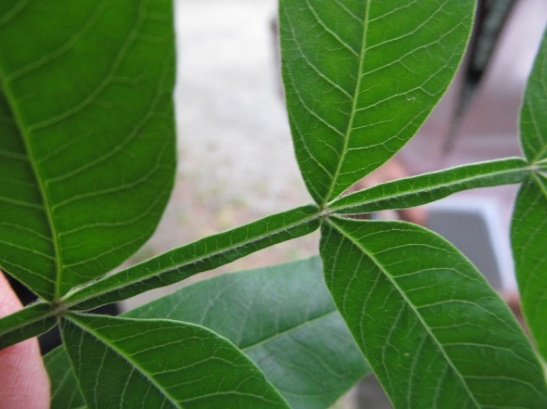
Crown and Leaves
The crown can be indicative of species at a distance: oval, round, pyramidal, weeping, taller than broad or broader than tall. Many tree field guides will have a sketch of the tree’s normal silhouette or a description of the geometric shape (as a lone tree).
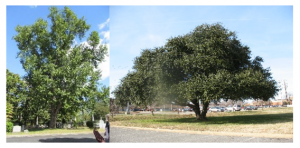
Leaves are arranged on their stems in alternate, opposite or whorled patterns. This is genetic, although variants may occur within a genus norm (as the Cornus alternifolia or alternate-leafed dogwood differs from its more common opposite leafed relatives Cornus florida et al). Whorls are much rarer but are a wonderful ID clue if found. The next most important distinction among leaves is whether they are simple or compound. This is a matter of looking for a bud in the place where the leaf or leaflet joins the next part of the structure (known as an axil). A leaf has a bud at that axil: it might be a swelling in the stem or a very obvious pointy bud, but there is an indication that this is a node. If you do not see such a node, go up to the next juncture, and so on.
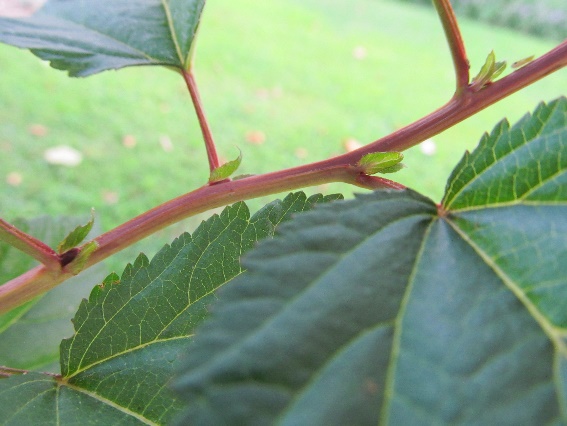
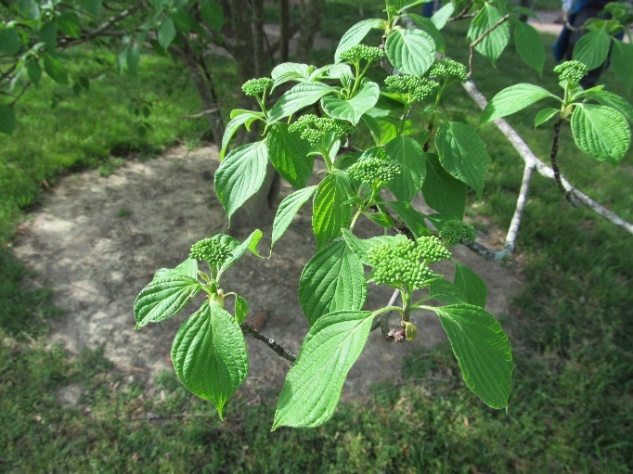
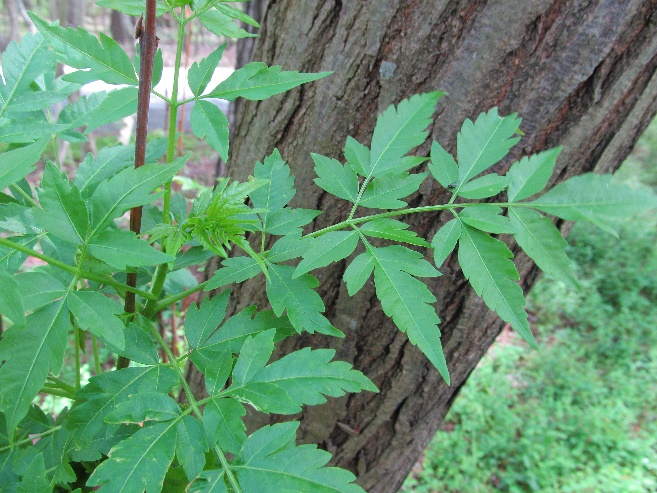
The example above of a compound leaf reveals another of the many variations possible among leaves. In this case, the entire ‘frond’ to the right is one leaf (bud at the axillary join with the twig), and the leaves are alternate. But the leaflets that make up the leaf are arranged oppositely. The devil is often in the details!
Beyond these first differentiations, the variety of possible leaves becomes very complex. Any competent field guide will have an introductory section with illustrations of leaf shapes, margins and veination (the pattern of how the veins are formed in the leaf).
Reproductive Systems
Generally, plants aim to reproduce themselves, so their strategies for making seeds are usually among the most varied and interesting of their behaviors. Flowers and fruits are only available for view in specific parts of the growing season (cones possibly longer), but when seen they can give particular insight into that tree’s larger pedigree. A good example is the rose family (Rosaceae), including apples, cherries and hawthorns, with sepals and petals in open groups of five. Chapter Five will provide much more detail on tree family relationships.
For gymnosperms, this means creating cones both male and female, which with wind pollination produce seeds. No flowers or fruit is needed, but the pollination process is quite wasteful of resources: witness the deluge of pine pollen every spring. For species identification, it is useful to know how many years the cones stay on the tree, as well as their size, shape and placement.
Ginkgos are not in either category, but rather a throwback to 190 million years ago, when there were many species of them all over the then-continents. Ginkgos have broad leaves but their ‘fruits’ are actually naked seeds with an outer fleshy layer, no flower involved. They differ from the gymnosperms in that the sperm swim a short distance in the fertilization process, which is an evolutionary throwback to ferns and mosses.[48]
Angiosperms are defined as “flowering plants” so this is an extremely broad topic. All angiosperm trees have flowers, though they may be very small and unnoticed. The American elm (Ulmus americana) has tiny flowers leading to small, flat green fruits with seeds in the very early spring. Many people do not even realize the elms even have flowers. In contrast, there are full scale festivals to celebrate the cherry blossoms just a month or so later. If we value our trees, we will understand their cycles and needs and plan accordingly.
A last word about tree identification: There are three main parts to the task. The most significant is surely to observe and be able to recognize the character and features of that species and perhaps even that individual tree. The next part is to learn about, or discover, relationships to other living things related to that species or particular tree. This means noting or looking for the other plants which typically grow well in company, as well as insects and birds which rely on those trees: in effect, what community is this tree part of? Lastly, there is knowing its accepted name: first, so you can communicate with other people about it; second, that you may learn something from the name itself. Common names may refer to a characteristic, such as the eastern red cedar’s rusty, shredded bark. Then the botanical name tells you where it falls in a taxonomic hierarchy: Juniperus virginiana is not even remotely a cedar, but actually a juniper.
There is a human response to the naming of a tree: Nancy Ross Hugo in Seeing Trees says “We take psychological possession of the things we can recognize. To me, getting to know a tree is like getting to know a human being—the more you know, the more the relationship deepens, and a person’s (or tree’s) capacity to surprise never ends.”[49]
Connections between Tree Physiology and External Factors
One of the most useful things to know about a tree species is where it originally came from, known as provenance. For example, Mediterranean trees tend to be tolerant of heat and drought, meaning that olives tend to do well in the US West, and their colder-hardy relatives (ash and fringe tree) have been good urban trees in the dry overbuilt cities of the temperate East and Midwest (at least until the emerald ash borer, another topic). Tropical trees grow without much seasonal variation and may deal with more abundant moisture, which may be reflected in root systems and wood grain. The soil of origin may hold clues to the preference for a preferred pH range, nutrient needs, and useful companion plants. As with the discussion of C3/C4/CAM photosynthesis, knowledge of the evolutionary conditions which produced a particular tree may help predict success in a challenging situation. It is also important to consider the factors which make native trees develop where they are. These factors include the habitat interface with insects and other animals (which may change under urban stress) and sun angle at latitude (which doesn’t change).
One of the big divides in trees is how they cope with freezing temperatures. In live xylem (trees not dormant or otherwise protected), freezing water can introduce air bubbles. Cavitation is the descriptive name for air bubbles in the conducting cells of the vascular system and can be very injurious to the tree.[50] The inability to protect from freezing limits the range of many plant species.
Trees in Groves and Forests. Most trees prefer to grow in company, at least in groves if not in forests. If they start out as small shoots together, or as shoots among a group of more mature trees, their stems will adjust to each other to share the available resources. Sun seekers will be thinner, straighter and taller as they reach for the sky.[51] Understory trees fill in and will stay relatively small, even shrubby depending on the species. The roots will tend to grow into a community arrangement with their attendant fungi. One result of a grove/forest growth pattern is collective stability in adverse weather conditions.
Alternatively, Lone Trees, which are set out to grow in the open, by themselves, will have broader canopies and thicker trunks than their forest cousins. Each such tree has to arrange for its own stability in wind and storms, meaning people must take care not to injure its naturally wide-spreading roots. If the lone tree is in a confined landscape, then extra care is needed to give this tree a chance. Understory trees planted in the open face a double challenge: adapting to more sun than they evolved for, and living an unnaturally solitary life. Plant breeders do make a wide variety of cultivars of particular favorites (for example redbud, Cercis spp), so it is worth researching the basic tree type and what differences the breeder has made. In general, maintenance programs for lone trees are more demanding, and more necessary, than for those in groves and forests.
Nursery trees grow in circumstances more like the lone tree, even though there may be a lot of them in one location. Either they are in containers or, if in the ground, their roots will be cut before they are dug up in order to have a manageable size root ball. Best practices call for making a part of this cut ahead of time so the cut roots can form new absorption roots within the ball: but the tree is still all alone. It will have to make up the lost root spread before it can really function properly in the landscape.
In a natural setting or urban park/large garden, a properly chosen tree’s genetic longevity should give time for it to adjust to being transplanted. However, the paved city environment and common street tree practices mean that even well-chosen trees often don’t have enough space and are subject to life-limiting threats such as poor soil, lack of water and the probability of mechanical assault (weedeaters, cars, etc). There are trees which are better suited to harsh conditions than others, and proper planning and care will also improve the odds of tree survival. Hence, the urban tree lover needs to (1) select wisely, (2) plant good quality nursery stock (3) in enough space or engineered soil, and (4) follow a plan of extended care sufficient to give the tree a chance to survive into maturity.[52] If such urban trees can be planted so their roots can mingle and their trunks and canopies can be mutually supportive, so much the better.
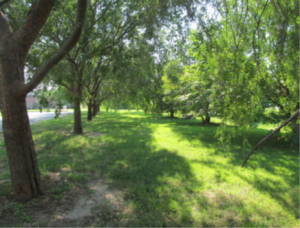
Finally, one last word about how it all fits together. The analogy of a baseball diamond is used here by permission of arborist Renee Frith, who has this explanation: If you (the tree) are the batter, you will need the bat, the ball, fast legs and all your skill to get to first base; then you will need energy reserves from photosynthesis to take you to second base; you will need to be properly hydrated to get to third base (especially if you steal it); and then if all goes well, you achieve your object and score the run by reproducing.
Happy Trees to All!
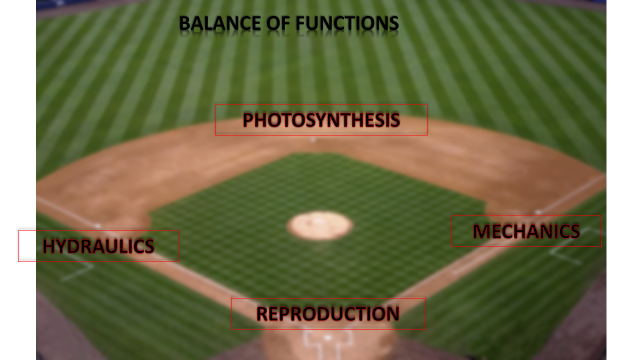
Review Questions
- What is the connection between photosynthesis, respiration and transpiration?
- Look around your home, garden, school or office: are there any C4 or CAM plants?
- Find a wooden board or table with visible rings (pine is great). Can you tell which direction was the bark/outer edge?
- Where are auxins made, and what are some of the things they do?
- Why are adventitious roots in dicot trees undesirable?
- Describe a good branch attachment structure.
- If a palm tree trunk is injured, can the tree compartmentalize the wound?
- In most Virginia settings, what are two advantages of planting trees in small groves versus alone in the landscape?
- Allaby, M. (2015). The Dictionary of Science for Gardeners. Timber Press. ↵
- Reece, J.B., Urry, L.A., Cain, M.L., Wasserman, S.A., Minorsky, P.V., Jackson, R.B. (2011). Biology (9th ed.). Benjamin Cummings. ↵
- Allaby, M. (2015). The Dictionary of Science for Gardeners. Timber Press. ↵
- Coder, K.D. (2012). Water and Trees: Understandings for Tree Health. University of Georgia, Warnell School of Forestry and Natural Resources Outreach Monograph WSFNR 12-11. ↵
- Capon, B. (1990). Botany for Gardeners. Timber Press. ↵
- Gilman, E.F. (2012). An Illustrated Guide to Pruning (3rd ed.). Delmar. ↵
- Pearcy, R.W., Troughton, J. (1975). C4 Photosynthesis in Tree Form Euphorbia Species from Hawaiian Rainforest Sites. Plant Physiology. www.plantphysiol.org. ↵
- Black, C.C., Osmond, B.C. (2003). Crassulacean Acid Metabolism Photosynthesis: ‘Working the Night Shift.’ Photosynthesis Research. 76, 329-341 ↵
- Reece, J.B., Urry, L.A., Cain, M.L., Wasserman, S.A., Minorsky, P.V., Jackson, R.B. (2011). Biology (9th ed.). Benjamin Cummings. ↵
- Dimmitt, M.A. (2017) Plant Ecology of the Sonoran Desert Region. Arizona-Sonora Desert Museum. www.desertmuseum.org. ↵
- Orcutt, D. (2016). How Do Plants Respond to Changing Climatic Conditions? [Lecture]. Virginia Master Gardener College, Norfolk, VA, United States. ↵
- Mattheck, C., Bethge, K., Weber, K. (2015). The Body Language of Trees (1st ed.). KS Druck GmbH. ↵
- Reece, J.B., Urry, L.A., Cain, M.L., Wasserman, S.A., Minorsky, P.V., Jackson, R.B. (2011). Biology (9th ed.). Benjamin Cummings. ↵
- Bergman, R., et al. (2010). Wood Handbook, FPL-GTR-190. Forest Products Laboratory, US Dept of Agriculture Forest Service. ↵
- Gilman, E.F. (2012). An Illustrated Guide to Pruning (3rd ed.). Delmar. ↵
- Missouri University of Science and Technology Lecture Notes. Wood Grain. Accessed at http://classes.mst.edu/civeng120/lessons/wood/wod_grain/index.html 10/15/2017 ↵
- Bergman, R., et al. (2010). Wood Handbook, FPL-GTR-190. Forest Products Laboratory, US Dept of Agriculture Forest Service. ↵
- Cassens D.L. (2007). Hardwood Lumber and Veneer Series: Elm. Purdue University Cooperative Extension. ↵
- Missouri University of Science and Technology Lecture Notes. (n.d.). Wood Grain. Missouri University of Science and Technology. http://classes.mst.edu/civeng120/lessons/wood/wod_grain/index.html ↵
- Bergman, R., et al. (2010). Wood Handbook, FPL-GTR-190. Forest Products Laboratory, US Dept of Agriculture Forest Service. ↵
- National Park Service. (n.d.). Bristlecone Pines. Great Basin National Park Nevada. https://www.nps.gov/grba/planyourvisit/identifying-bristlecone-pines.htm ↵
- Harris, R.W., Clark, J.R., & Matheny, N.P. Arboriculture. Fourth Edition. Upper Saddle River NJ: Prentice Hall; 2004 ↵
- Rindels, S. (1995). Sidewalks and Trees. Iowa State Extension Horticulture and Home Pest News, 3-31. ↵
- Gilman, E.F. (2017). Tree Root Growth After Planting [Power Point Presentation]. Hort.ifas.ufl.edu. ↵
- Urban, J. (2008). Up By Roots. International Society of Arboriculture. ↵
- Gilman, E.F. (2012). An Illustrated Guide to Pruning (3rd ed.). Delmar. ↵
- Gilman, E.F. (2017). Tree Root Growth After Planting [Power Point Presentation]. Hort.ifas.ufl.edu. ↵
- Harris, R.W., Clark, J.R., Matheny, N.P. (2004). Arboriculture (4th ed.). Prentice Hall. ↵
- Gilman, E.F. (2012). An Illustrated Guide to Pruning (3rd ed.). Delmar. ↵
- Mattheck, C., Bethge, K., Weber, K. (2015). The Body Language of Trees (1st ed.). KS Druck GmbH. ↵
- Ali, A.D., Burkhart, L. Jr. (2017). Palms: Woody Giants of the Monocots. Arborist News. 26(4): 12-20 ↵
- Capon, B. (1990). Botany for Gardeners. Timber Press. ↵
- Harris, R.W., Clark, J.R., Matheny, N.P. (2004). Arboriculture (4th ed.). Prentice Hall. ↵
- Reece, J.B., Urry, L.A., Cain, M.L., Wasserman, S.A., Minorsky, P.V., Jackson, R.B. (2011). Biology (9th ed.). Benjamin Cummings. ↵
- Allaby, M. (2015). The Dictionary of Science for Gardeners. Timber Press. ↵
- Appleton, B. (2015). The Walnut Tree: Allelopathic and Tolerant Plants. VCE Publication 430-021. ↵
- Dujesiefken, D., Liese, W. (2015). The CODIT Principle: Implications for Best Practices. International Society of Arboriculture. ↵
- Gilman, E.F. (2012). An Illustrated Guide to Pruning (3rd ed.). Delmar. ↵
- Gilman, E.F. (2012). An Illustrated Guide to Pruning (3rd ed.). Delmar. ↵
- Mattheck, C., Bethge, K., Weber, K. (2015). The Body Language of Trees. (1st ed.). KS Druck GmbH. ↵
- Gilman, E.F. (2012). An Illustrated Guide to Pruning (3rd ed.). Delmar. ↵
- Reece, J.B., Urry, L.A., Cain, M.L., Wasserman, S.A., Minorsky, P.V., Jackson, R.B. (2011). Biology (9th ed.). Benjamin Cummings. ↵
- Gilman, E.F. (2012). An Illustrated Guide to Pruning (3rd ed.). Delmar. ↵
- Harris, R.W., Clark, J.R., Matheny, N.P. (2004). Arboriculture (4th ed.). Prentice Hall. ↵
- Harris, R.W., Clark, J.R., Matheny, N.P. (2004). Arboriculture (4th ed.). Prentice Hall. ↵
- Gilman, E. F. Landscape Plants: Tree Structure Basics: Structural Defects: Hollows and Cavities. University of Florida Extension. http://hort.ifas.ufl.edu/woody/Cavity.shtml ↵
- Iowa State University Forestry Extension. (n.d.) Tree Identification: American Basswood. Iowa State University Forestry Extension. https://www.extension.iastate.edu/forestry/iowa_trees/trees/basswood.html ↵
- Crane, P. (2013). Ginkgo: The Tree that Time Forgot. Yale University Press. ↵
- Hugo, N.R. (2011). Seeing Trees. Timber Press. ↵
- Gilman, E.F. (2012). An Illustrated Guide to Pruning (3rd ed.). Delmar. ↵
- Gilman, E.F. (2012). An Illustrated Guide to Pruning (3rd ed.). Delmar. ↵
- Urban, J. (2008). Up By Roots. International Society of Arboriculture. ↵